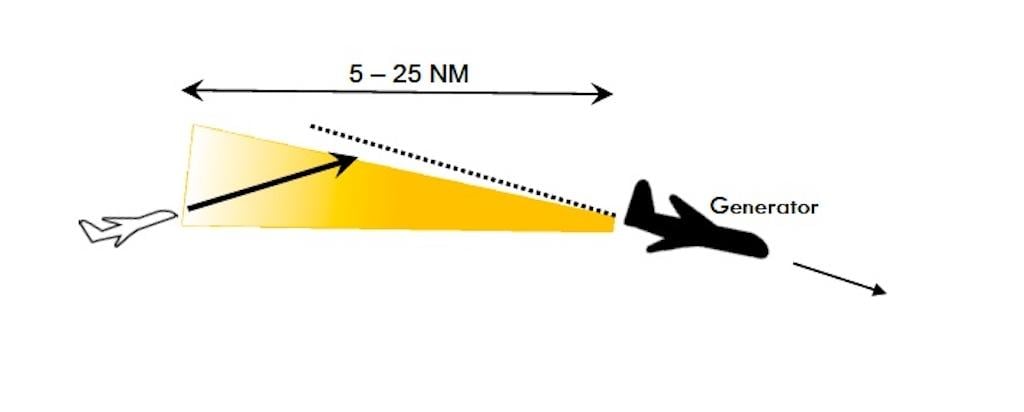
Wakes can be encountered up to 25 nm behind the generating aircraft. The most significant encounters are reported within a distance of 15 nm.
Fast cruising speeds at high altitude create another direct risk factor for high-altitude wake turbulence encounters. During a “cross track” encounter, fast true airspeeds create larger induced load factors on an aircraft that can be outside of the aircraft’s structural flight envelope.
An additional risk factor is that enroute wake turbulence encounters occur without warning and when occupants aren’t securely belted in their seats. This elevates the degree of potential injury to levels in which severe cerebral-spinal injuries are likely during impacts with the upper cabin.
‘Clean’ Wings And Vortices
The initial strength of trailing vortices depends upon the weight, wingspan, angle of attack, configuration and speed of the airplane. There are substantial differences in the strength, motion and duration of wake vortices created by a clean wing versus a “dirty” wing.
Look at some of the photos taken of aircraft on approach in humid atmospheric conditions that illuminate the twin vortices. Depending on each aircraft’s unique wing and flap design, you will notice on some aircraft that the twin vortices are coming from the outboard section of the flaps, not the wing tips.
There actually is a reason why this becomes important to an understanding of wake turbulence. A “dirty” wing (with flaps extended) produces a series of smaller vortices from the outboard section of each major flap section as well as at the wing tip. These imbedded vortices interact with each other and create some turbulence within the overall vortex. This lessens the strength of the overall vortex.
In contrast, a clean wing produces a single coherent vortex. The general lack of turbulence within a coherent vortex formed from a clean wing will tend to produce longer lasting vortices.
Wake Vortex Motion
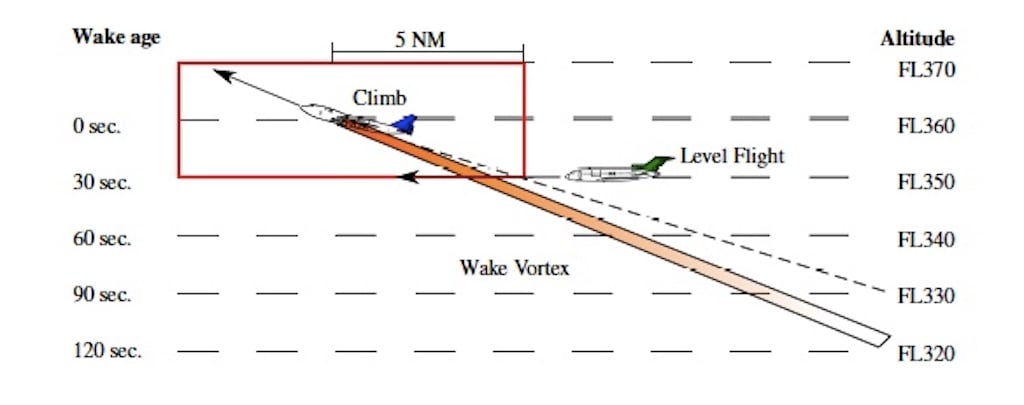
Avoiding an encounter with wake turbulence relies on accurately predicting its location and strength. On average, vortices sink at about 400 ft./min while drifting with the wind. But wake vortices actually exhibit significant variation in their behavior.
According to Claude Lelaire, who conducted the largest in-flight study of aircraft behavior in wake turbulence as head of the Airbus flight test department: “Depending on weather conditions the descent rate may vary significantly and may even be very small. One of the key factors affecting this descent is the variation of the temperature with the altitude. A temperature inversion limits the rate of descent.”
What might contribute to this variation in descent rate? During descent, the wake is compressed by increasing atmospheric pres¬sure, which in turn increases the temperature of the wake. The net warming of the wake then creates a buoyancy effect which in certain temperature conditions can cause the wake to remain at a constant height rather than descend.
Post-incident analysis of some high-altitude wake encounters has revealed wake vortices that apparently moved downward at an increased descent rate. Currently there isn’t enough research to precisely determine how much vertical downdrafts or another phenomenon are responsible for this increased descent rate, but it happens and it contributes to this variation in wake motion.
Vortex Dissipation
On average, the rate of decay lasts between 2-3 min. Videos taken during high humidity conditions reveal that sometimes the two vortices exhibit significant arching and bending, unlike posters and presentations which give the impression that the trailing vortices predictably descend.
In short, there is a lot of energy enveloped in those tight cores. Vortices with high rotational speed will begin to develop an instability in their motion. You can see this for yourself on days when a jet is leaving a contrail. The two vortices will develop waviness in their motion until the vortex lines almost touch. The vortices then pinch off and reconnect into a line of vortex rings that slowly dissipate. This is called the Crowe Instability.
During this transformation, the energy of the vortices is rapidly re-aligned. An aircraft flying through this period in a wake’s existence is more likely to experience generalized turbulence with random aircraft motions rather than a strong roll.
An in-depth research report titled An Improved Understanding of En-Route Wake Vortex Encounters, conducted by the engineering department at the Delft University of Technology and Eurocontrol, found there is an atmospheric region with low vertical stability approximately 3000-5000 ft. below the tropopause that creates an elevated risk for wake turbulence encounters due to a combination of temperature and lapse rate, which enhance the persistence of wake vortices. Wake vortices generated by aircraft in enroute conditions have been reported to last up to three minutes.
It is important to note that the tropopause is not located at one constant altitude. The tropopause altitude varies between days and locations, and is influenced by weather systems. Its estimated altitude can be found on meteorological charts.
How to be prepared in the event of a wake turbulence incident, in Part 3 of this article.
Encountering Wake Turbulence At High Altitude, Part 1, https://aviationweek.com/business-aviation/safety-ops-regulation/encountering-wake-turbulence-high-altitude-part-1