実証試験が近付く中、極超音速技術のハードルに直面する研究者 | Researchers Face Tougher Hypersonic Barriers As Weapon Tests Loom

数十年にわたり到来すると言われ続けてきた、実用的な極超音速機の時代がようやく幕を開けようとしている。かつてはSFや軍事プランナーの空想の産物とされていた概念が現実になりつつある。
アメリカでは第1世代の戦術ブーストグライドおよびエンジン搭載型の攻撃兵器が開発中で、数年以内には配備される。しかし、推進派であっても、攻撃・偵察・民間および軍用の高速輸送・宇宙への多段式移動手段といったより幅広い用途や、マッハ5以上のスピードを有効活用できる次世代技術や大型化に向けては、まだまだ多くの課題が残されていると考えている。
研究者は、推進方式の選択や、推進装置と機体の最適なインテグレーションといった一次的な問題から、マッハ数の変動に伴う流体力学の変化による困難な問題などにも対処する必要がある。これほどの高温に対応できる夢のような耐熱素材も未だ発明されておらず、ハードルのリストは相変わらず埋め尽くされている。
しかし、ロケットブースターで加速される極超音速滑空体や、初期のスクラムジェット実証機は、今日の開発者に対して高速域における基礎的な空力特性や推進力に関する要件などについて、確固たる発見を与えることができた。国防総省で近代化研究開発のディレクターを務めるMark Lewis氏は「グッドニュースは、差し引きでプラスの推力を生み出すスクラムジェットは、間違いなく製造可能だということだ」「これは大きな成果だ。我々は燃料の特性がどのようなものであるべきか、そしてどのように噴射するのが効率的かを明確に理解している」と述べた。
しかし、使い捨てのロケット推進型グライダーやミサイルの先にある、再使用可能な極超音速機を開発するという究極のビジョンを実現するためには、まだまだ膨大な労力が必要になるとルイス氏は語る。「エンジンと機体のインテグレーション、特に推進力の切り替えの部分に多くの研究が必要だ」「マッハ0からマッハ5~6に加速し、そこから0に戻せる推進システムが必要になる。今この問題に取り組んでいるところだ。ここで浮かぶ疑問は、これを複合サイクルエンジンにするべきか、それとも複数のエンジンを搭載するべきなのか?そして、タービン推進から直接ラムジェットやスクラムジェットに切り替えればいいのか、それともその間に何かが必要になるのか?」と同氏は説明した。
タービンエンジンの作動限界がマッハ3~4あたりであることから、エンジン開発者がマッハ5以上まで加速させるための方法として注目してきたのが、高速ターボジェット・ラムジェット・スクラムジェットの組み合わせ(総称してタービンベース複合サイクル:TBCC)だ。しかし、スクラムジェット推進のNASA X-43Aや空軍主導のX-51A、そして推力13,000ポンドを発揮するノースロップグラマン社製スクラムジェットの空軍による2019年の試験成功を受けても、統合型高速推進のための最善の方法について決定的な答えは出ていない。
その理由のひとつは、スクラムジェットは可動部分がなく概念的には単純であるが、作動原理は複雑だということだ。ジェットエンジンにおける機械的な圧縮は、エンジンに入ってくる衝撃波の圧縮に置き換えられる。ここでは化学反応を伴う乱流現象が発生する前に、インレットとアイソレーターの中で空気を圧縮し、1ミリ秒以下で燃料と空気を反応させる必要がある。
実際に運用された吸気式のエンジンとしては最高速を発揮した、プラット&ホイットニー社製J58ターボジェットに搭載されている50インチのコンバスターが1960年代に達成した性能を基準にしても、スクラムジェットに与えられた役割は桁違いに困難なものだ。ロッキードSR-71に搭載されたJ58は高度80,000フィートをマッハ3.3(毎秒3,200フィート)で飛行したが、X-43Aの30インチのコンバスターは高度110,000フィートをマッハ9.7(毎秒9,600フィート)で飛行した。
X-51Aの概念設計を主導した、ボーイング社の極超音速技術担当チーフサイエンティストのKevin Bowcutt氏は次のように述べた。「難しいのは、コンバスター全体の最適な位置関係(容積および形状)、インジェクターおよびフレームホルダーの位置関係、燃料インジェクターの配置、さらにその他インジェクション関係のパラメーターの調整などだ」。彼は、このハードルは高いものの、空力・制御・推進・構造といった考慮事項を同時に組み合わせて高度に統合された解決策を導き出す、学際的な設計最適化(Multidisciplinary Design Optimization:MDO)アプローチを用いることで対処することができると考えている。
「この設計最適化プロセスは、高速度で忠実度の高いフローシミュレーション(数値流体力学)ツール、極超音速エンジン試験に特化した試験施設、試験計測のための精密な診断機器によって支援される」と付け加えたのは、以前X-51AがMDOを用いて設計が洗練された最初の極超音速機であることを指摘した、Bowcutt氏だ。
しかし、何十年もスクラムジェットの開発が進められているにも関わらず、これに代わる推進システムのコンセプトは生まれ続けている。果たして、スクラムジェットを用いることは今でも正解なのだろうか?Lewis氏は「その答えはまだ出ていない、と言っておこう」「我々の課題のひとつは、もしかすると高速性を実現できる他の方法があるかもしれないと、常に心を開いて考え続けることだ。テクノロジーの選択肢を狭めるようなことはしたくない」と話した。
推進システムの選択肢
静止状態の機体をマッハ6以上まで加速させるためのTBCCに代わる可能性を持った推進システムは幅広く研究されている。その多くは冷却と動力のための作動流体として用いられる液体水素の熱力学的特性を利用したものだ。たとえば、JAXAが研究しているエキスパンダーサイクルを用いたエアターボ・ラムジェット(ATREX)や、ロシアが開発した深冷処理を用いるエアターボ・ロケットATRDC、熱統合深冷ターボジェットおよび液体ロケットエンジンを用いるKLINサイクル、そしてアスピレーションロケットエンジンだ。
その他の見込みがある吸気式エンジンのコンセプトとしては、回転/パルスデトネーションエンジンや、さらに高度な磁気流体力学/磁気プラズマ力学エンジンなども可能性を持っている。両方とも極超音速機への活用が研究されているが、前者にはパルスデトネーションロケットをベースに、エジェクターラムジェット・ラムジェット・スクラムジェット・ロケットモードを組み合わせた複合サイクルの一部として、宇宙飛行を実現する可能性もある。
もうひとつの選択肢は、Reaction Engines社のSABRE(Synergetic Air-Breathing Rocket Engine)だ。これはイギリスとアメリカの拠点において開発中で、吸気モードでは最大マッハ5.4を発揮し、ロケットモードでは機体を軌道速度まで加速させることができる。10月に行われた試験で、このエンジンのプリクーラーはマッハ5相当の吸気温度で正常に作動し、重要なマイルストーンを達成した。
「液体エアサイクルエンジンや、深冷エアサイクルエンジンの考え方は非常に有望だ」「最終的にそれらが形になるかは分からないが、いくつかのクラスの機体には非常に大きなメリットがあると考えられる。私が心配しているのは、幅広い優れたアイデアが生まれる余地を残すためには、充分に多様な研究および開発ポートフォリオを維持しなければならないということだ」とLewis氏は語る。
機体と推進装置のインテグレーション
吸気型エンジン搭載機は速度と高度が上がるにつれ、推進力を維持し続けるために比較的狭い回廊の中を飛行する必要がある。マッハ3前後でタービンエンジンから切り替わり、炭化水素を燃料とするスクラムジェットはマッハ7.5前後で燃料の熱限界に達し、水素燃料の場合はマッハ14あたりで性能限界を迎える。高度に関して言えば、大半のデュアルモードスクラムジェットは理論上、動圧500psfあたりが限界で、これは100,000フィートでマッハ5、150,000フィートでマッハ15程度に相当する。
低高度では大気の密度が高いことが別の障壁になっている。通常、機体設計者は成層圏内での極超音速飛行を念頭に、動圧を2,000psf以下に収まるよう設計している。結果として、構造設計上の限界を表すラインは40,000フィートでマッハ2.5の点と110,000フィートでマッハ14の点を結ぶ形になる。
これらの課題に加え、マッハ数によって変動する空力特性も設計の検討をさらに複雑なものにする。たとえば、変化する飛行モードに最適な翼面積を見つけること、高い質量分率を持つ推進剤に合わせた機体の構成、マッハ数によって大きく変わる適切なインレットおよびノズルサイズの定義などだ。
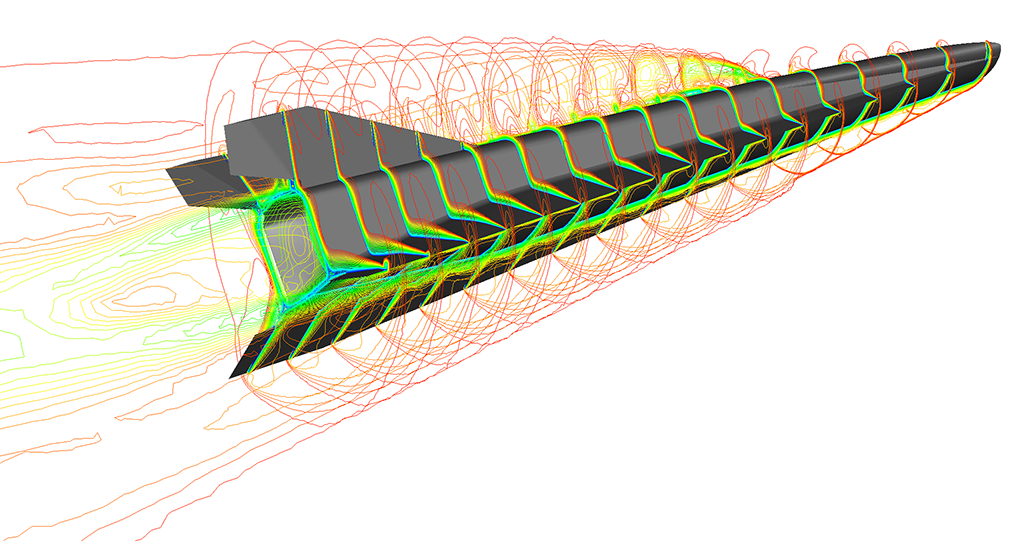
Lewis氏は「我々は良いインレットの作り方も知っている」「ただ、現在進行中の疑問もある。それは、『最高のインレットの設計とはどんなものか?』 だ。『完璧主義は”必要充分”の敵である』という格言の下で、我々は正しく機能するインレットを作ることはできる。ただし、より多くの開発・研究・改善の余地があることも明らかだ」と語る。
マッハ5以上を実現するための効率的な加速には高い推力比が鍵となるため、推進システムのインテグレーションが不可欠であり、マッハ数に応じて空気取入量を増やす必要がある。設計者は、全ての速度域で2以上の推力比を得るためには、全体的な空気取入量とエンジンの推力係数が充分に高くなくてはならないことを発見した。しかし同時に、極超音速性能を発揮するためにはインレットのサイズと空気取入量は比較的大きくする必要があるが、機体が遷音速域を突破するためにはインレットやノズルによる抵抗は減らさなくてはならない。
「マッハ数・高度・迎え角が大きく変動する中で、高い圧縮効率と気流の安定性、少ない気流乱れと付随する抗力(流出を含む)の少なさという特徴を備えた、幅広く変化する空気取入量(取入面積として測定)と収縮比に対応するインレットを設計することは非常に困難だ」とBowcutt氏は述べた。
「長年にわたり、私は多くの極超音速機の概念設計に関わってきたが、最終的な形状は極超音速域における性能ではなく、遷音速域の性能により決定されてきた」とLewis氏は回想する。「マッハ5、6、7、8で優れた性能を発揮する極超音速機の形状を設計することはできるが、それではマッハ1を突破することができない。これが兵器であればより簡単で、たとえばロケットなどでマッハ1を突破させることができるからだ。これが低いマッハ数における性能が重要になることを示している」とも付け加えた。
機体と推進システムを密接に統合させるという極めて困難な課題は、機体の重量および機内サブシステムの動力や容積を最小限にする必要性によって、さらに増幅される。MDOのようなアプローチによって可能になった、高度にインテグレーションされたフライトアーキテクチャの必要性は「絶対的に重要な分野だ」とLewis氏は語る。「35年前、極超音速機は完全に統合されたシステムである必要があることは分かっていたが、今日我々が学んだことの全てがこの考えを補強した」とも話した。
大型化と流体力学の関係性
機体のサイズによっても難易度が違ってくる。メリーランド州にあるジョンズホプキンス大学応用物理学研究所の航空・ミサイル防衛部門長を務めるDavid Van Wie氏は「大型機になると、機体が柔軟な構造であることも非常に重要なため、結合方法という新たな問題も出てくる」「ただ頑丈にすればいいというわけにはいかず、結合が強すぎると機体にかかる荷重がエンジンや推進装置に戻ってくることがあるため、機体が大型になっていくほど結合の程度は難しい問題になってくる」と語る。
小型の機体は構造的には頑丈なものの、パッケージングや熱処理の問題に直面する。Van Wie氏は「だいぶ昔のNASAの研究によると、短くずんぐりした機体よりも、細長い機体の方が極超音速域における揚力比がはるかに大きい」「そして、何か小さいものを作ろうとしたとき、それを細長くすると内部容積が足りなくなる。結局、揚力比から決まる空力効率により、その中で実現可能な性能が設定されてしまう」と話す。
「機内のパッケージングを考慮しつつ空力と推進装置のインテグレーションを行うことにも課題がある」「誘導・航法・制御に必要なセンサーやシステムといったパーツを搭載できるスペースを残しておかないといけない」とLewis氏は付け加えた。
大型化に伴うさらなる問題は、極超音速機設計の核心ともいえる、境界層の特性に関わる問題だ。これは表面近くにある薄い層で、設計者はなるべく長い時間この層流を維持することで発熱を最小化し、必要な熱対策を減らすべく苦心している。極超音速域では境界層は厚くなる傾向があり、より外乱への耐性が高まる。しかし、これが乱流に移行すると発熱・抵抗・安定性に影響を及ぼすことがある。衝撃波による圧力上昇も境界層との干渉を招く。
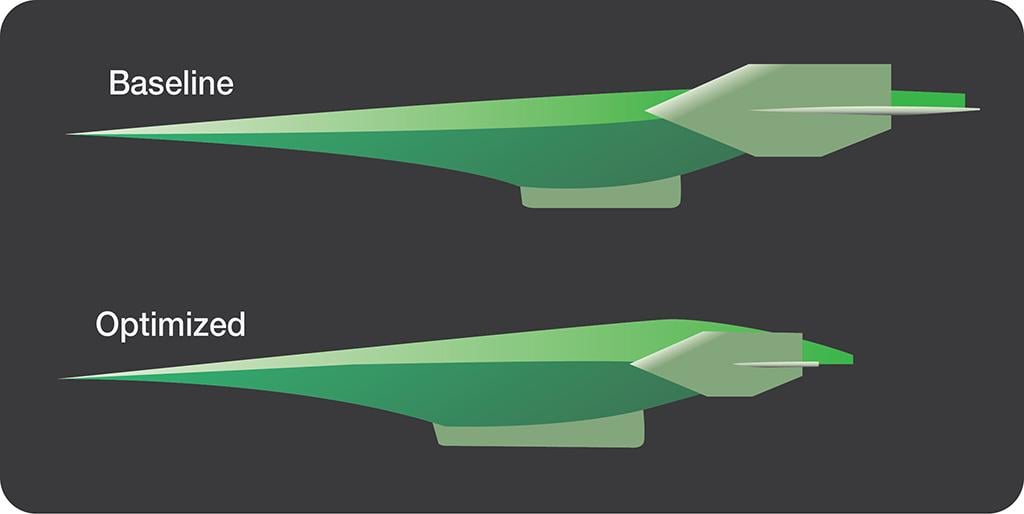
衝撃波位置が変わること自体は問題ではないが、衝撃波と境界層の干渉は摩擦抵抗を発生させ、場合によっては層流の8倍もの空力加熱が生じることもある。衝撃波位置が変われば、干渉するゾーンも変化する。「このため、空力加熱が大きくなる範囲は常に移動し、これにより機体の多くの範囲で熱対策または熱管理が必要になる(そして重量とコストも増加する)」「境界層の遷移を正確に予測することが重要だ。境界層の遷移を遅らせる手段が開発でき、それが使用可能になるのなら、これも重要なものになってくる」とBowcutt氏は説明する。
最近ではコンピューターコードの進歩により流体力学のより正確な予測が可能になったが、境界層の遷移ゾーンをさらに絞り込むことができれば、極超音速機設計をより堅実なものにできるとLewis氏は語る。「我々は、”我々がまだ分かっていないことを分かっている”ものを設計する方法を学んできた」「境界層の遷移がいつ起きるかを正確に知ることは永遠にないと気付いたので、それを踏まえて設計する必要がある」とも付け加えた。
逆にスクラムジェットエンジンの開発者としては、作動性を向上させるためにインレットの境界層の状態を層流ではなく乱流にしたいという問題がある。「乱流の方が、衝撃波が境界層と干渉した際の境界層分離の影響を受けにくくなる」とBowcutt氏は話す。
1980年代の国家スペースプレーン(National AeroSpace Plane:NASP)プログラムで学生として働いていた時に学んだことを思い返すと、もし機体の境界層が層流ではなく乱流だった場合、その機体は絶対に飛ばないと年配の講師が警告していたとLewis氏は振り返る。「私がここから学んだことは、もし我々の機体設計がそれほど敏感なものであれば飛ばない。よって堅実な構成で設計する必要がある。それが難しいところだ」と彼は語る。
極超音速機を一般的に運用可能なシステムにまで成熟させることは、依然として高いハードルであることをVan Wie氏も認めている。「実際にどのように機体を製造し、それを環境に合わせて着実に運用することの知識を向上させることは、今ある中でも大きな課題だと思う」「与えられた試験飛行の条件のように、針の穴に糸を通すような飛ばし方ではなく、そのような制約なしに他のあらゆる航空機と同じように飛ばしたい」と彼は話す。
また、特に高高度における細長いブーストグライド機や鋭角形状の再突入機では、機体の先端付近の衝撃波と境界層の干渉も問題になる。流れの干渉効果、つまり先端部の粘性相互作用がどのように発生するかは、レイノルズ数(慣性力と粘性力の比である無次元スケーリング測定法)の影響を受ける。レイノルズ数が小さい高空では境界層の厚みが増し、隣接する流れと干渉する。たとえ極超音速機の非常に鋭角な先端部であっても、非常に小さいレイノルズ数では境界層の肥大化は先端部の形状変化を招き、境界層の外側にある対抗流に対して鈍角であるように作用する。
この効果が先端部分の空気分布を変化させることで境界層の性質に影響を及ぼし、揚力/抗力に悪影響を与える可能性がある粘性相互作用を生じさせる。同様の効果はインレットやインレットの傾斜部における衝撃波/境界層干渉においても問題を起こす可能性がある。「これは先端部の性質に関する重要な要素だが、干渉が変化する度合いを教えてくれる」「極超音速下において、ある性能を発揮する形状を写真的に縮小してみると干渉の仕方が変化することから、突如としてまったく違う物理的な性質を捉えることができる」とLewis氏は語る。
高性能耐熱素材
素材についても大きな課題がある。宇宙船・弾道ミサイル・超音速機などに用いられている熱防護システム(Thermal Protection Systems:TPS)やその他の熱対策につながった70年にわたる研究にも関わらず、大気中における長時間の極超音速飛行に特有の熱問題に対処できる素材は存在しない。
マッハ6以上での継続的な飛行においては、極超音速機の先端部分は1,600~2,200℃となり、その上限はチタンの融点より300℃高く、鉄の融点より600℃高い高温となる。また熱流束の最高点は比較的小さな面積にブロートーチのように収束するため、高い熱衝撃と極端な熱勾配をもたらす。これほどの高温になると飛行中に素材特性が変化することもあり、酸化や触媒効果も起こる可能性がある。
Van Wie氏は「これは発明の機会がたくさんある領域だ」「先端部が鋭角になるほど、高温になりやすくなる。これが素材に関する問題のひとつだ。どこまで鋭角にしたいかによって、その機体が飛行できる最高温度が決まるといえる。その答えは、再使用する機体なのか使い切りの兵器なのかによっても変わってくる。これは活発に研究されている分野だ」と話す。
また同氏は、「より高い温度に対応した耐熱素材は、より鋭角な先端部を持つ設計を可能にし、それはより高い推力比による高性能を実現することにつながる。あるいは、より高速で高高度での飛行を可能にすることでマージンを確保することもできる」「これは改善の余地が多く残されている、チャレンジングな領域のひとつだ」と語った。
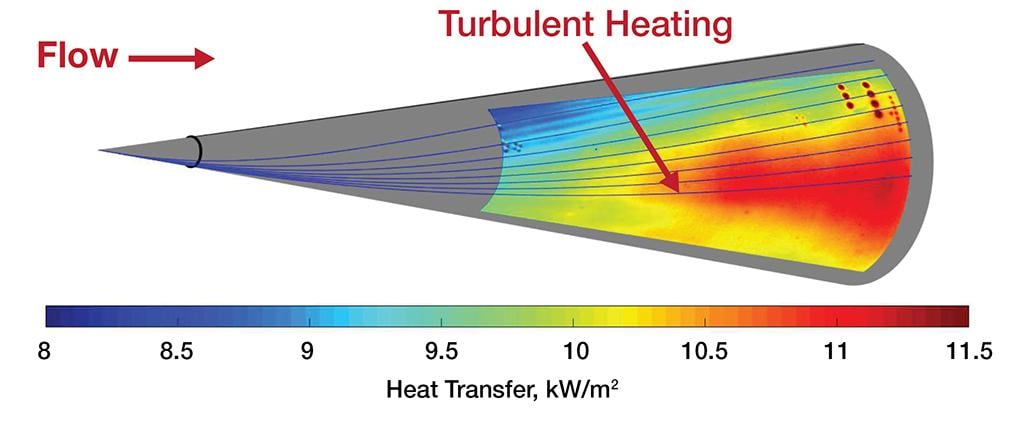
これに関連するもうひとつの重要なハードルは、機体サイズの変化による熱環境の変化だ。「機体サイズによって 素材の課題がどのように変化するかはまだ分かっていない」とLewis氏は述べた。「これは継続的な投資と開発が必要なもうひとつの問題だ。たとえば、ある極超音速機の構造を写真的に縮小すると、結果としてはるかに鋭い先端部ができあがる。ということは、これははるかに熱くなるということだ。そのため、結局は抗力が大きい鈍角の先端部による低い空力性能とのトレードオフになってしまう」
「特にまとまった数の極超音速機を生産するという話であれば、これは活発に議論される分野だ。大量生産にあたっては、ある機体サイズでうまく機能した設計であっても、それを幅広いファミリー機に応用することには細心の注意を払う必要があることに気付いた」と彼は付け加えた。
マッハ5以上で大気中を飛行すると、空気そのものが変化することがある。「温度が上昇すると、機体の周りを流れる空気の化学組成が変化し始める」「まず酸素が解離し始める。さらに高温になると、今度は窒素が解離を始める。この解離現象が機体の周りを流れる空気の特性に影響を及ぼす」とLewis氏は語る。二原子酸素の酸素ラジカルへの解離は約1,700度で発生し、窒素の場合は約3,700度で発生する。
高いマッハ数や空力加熱により引き起こされる解離現象やプラズマ形成は、極超音速機との通信や誘導・航法・制御(Guidance, Navigation and Control:GNC)を行う上で大きな問題となっている。「これは極超音速域の計算をやや困難にする。センサーをどこに配置し、必要な開口部や窓をいかにして機体に溶け込む形に整形すればよいのか?」とLewis氏は問いかける。
GNCの課題に対処するための研究開発は極超音速事業全体で活発に行われており、現在の研究ではミサイルや大気圏外往還機向けの新しいセンサーやアビオニクス、半自律/自律誘導式ミサイル誘導システム向けの先進的な設計、高速機の軌道最適化のための新しい誘導法の開発に至るまで、幅広い研究が行われている。その他の研究分野としては、目標選定や追尾アルゴリズムへのデータフュージョンの応用、新たな適応制御アルゴリズムや新型のアクチュエーターシステムの開発といったものがある。
高度な素材は、極超音速機の開発において「最初にして最重要の」課題であると、ロッキードマーチン社Missiles and Fire Control部門の技術担当ディレクター・ David Hunn氏は語る。同氏は「現在入手可能な素材システムの限界に基づいて、機体の軌道と性能を調整せざるを得ない」と、11月にロンドンで開催された王立航空協会の極超音速会議で講演している。
「我々は、これまでほとんど探索されてこなかった領域の中にいる。それにどのように対処するべきか?」と彼は問いかけた。選択肢は、循環するクーラントを用いた内部冷却構造から、パッシブ/アブレーティブ/セミアクティブTPSによる断熱構造まで多岐にわたる。しかし、全体的には放射冷却/高温構造が有力視されている。「ポンプなどの可動部がなくシンプルで、予測可能で安定もしているからだ」と彼は話す。「また、おそらくこれが最小のサイズ・重量・消費電力を実現することになるだろう」と付け加えた。
コーティング(表面処理)の課題
機体が受けることになる熱流束に金属では耐えられないため、現在のところ素材の選択肢は主に炭素繊維・炭素マトリックス複合材・セラミック複合材が中心になっている。
「我々は適切な素材が存在するという希望を持っているが、しかし…いつも『しかし』が付いてしまうが、炭素系素材は酸化してしまうということを理解する必要がある」「空気中では触媒反応により空気が元素に分解され、それが機体と再結合することを考慮する必要がある。そしてこれは吸熱反応なので、さらに熱が加わることになる。また、熱放射率についても考えておく必要がある。これが低すぎると高温になりすぎてしまう。基本的には、例えどんな素材で機体を作ろうとも、このような環境の下でも機能する保護コーティングを開発するしかないだろう」とHunn氏は語る。
1,700℃を越える温度に対応する新しいコーティングの研究は、タンタル・ハフニウムカーバイド・ジルコニウム・ハフニウムホウ化物などの新しいセラミック材料を中心になされている。「一部はセラミックがベースの素材なので、優れた酸素バリア性を持っている。このことから、現在の極超音速機にとっての短期的な解決策として、これら先進的な複合素材の上に新しいコーティングを施す研究が盛んに行われている」とHunn氏は述べた。
しかし、極超音速機の開発を次のレベルに進めるためには「母なる自然を無視して、我々自身で独自の素材を開発しなくてはならない、というのが答えだ」と彼は話す。大学や研究所では「極超音速技術に必要な特性を持つ新たな素材を考え出す」ことに重点を置き、コンピューターや周期表のロードマップを用いた基礎的な研究が進められている。
中国とロシアの研究成果を見ると「彼らは新素材を開発し、それを極超音速域に適応させるという点において、最先端の技術を大きく進歩させている」とHunn氏は話す。たとえば、モスクワにある国立科学技術大学の科学者達は最近、極超音速機の尖った先端部やノーズコーンにかかる典型的な熱負荷である2,000度に耐えられるという、新しいセラミック化合物の試験が行われたことを明らかにした。ハフニウム炭窒化物と呼ばれるこの新素材の融点はまだ不明だが、4,000度以上までの試験が行われていると科学者達は述べている。彼らによれば、極超音速域での試験も計画されている。
外部加熱の問題は、グライダーであれエンジン搭載機であれ、高密度に機器が詰め込まれた極超音速機内部のエンジニアリングや設計をする上で課題になる。「外部の表面は高温になろうとし、内部には高温が許されない電子機器がある」とVan Wie氏は話す。
この問題は、機体外部からの熱を遮断するために組み込まれている断熱材によってさらに複雑化する。「内部で生まれた熱流束はどうすればいいのか?」とVan Wie氏は問いかける。「その問題に不思議なことは何もない、これはただのエンジニアリング上の課題だ。これに対処するには様々な方法があるが、システム目線で見て、それぞれの機体のタイプや用途に合わせて最適な方法を考える必要がある」と彼は述べた。
生産体制の強化に備えて
特にアメリカの第1世代極超音速兵器の開発者にとって、最後の、そして緊急の課題は、予定されている生産拡大を支える産業基盤の確立である。今年初め、国防総省の調達部門幹部は業界のサプライチェーンの強みと脆弱性を評価するために「ウォールーム」を設置した。
X-51Aのスクラムジェットを開発した、推進システムに強みを持つAerojet Rocketdyne社は、DARPAのGlide BreakerやOperational Firesプログラムから、TBCCの推進システムであるAdvanced Full Range Engine、ロッキードマーチン社と共同開発しているHypersonic Air-breathing Weapon Concept(HAWC)に至るまで、幅広い攻撃用・防衛用双方の極超音速兵器プログラムに積極的に参画している。
Aerojet Rocketdyne社の防衛事業担当上級副社長・Tyler Evans氏は、「我々は受注したプログラムを実行し、いざという時に必要とされる能力とキャパシティを持つことに重点を置いている」「 X-51は超音速環境下における燃焼科学が実用可能かを検証する実証機であり、それは実現した。そして10年後には、我々はスクラムジェットをより一般化し、再使用を可能にし、コストを下げることに注力している」と語った。
生産体制を強化していくにあたり、同社は添加剤の製造に強みを持ち、フロリダに拠点を置く3D Material Technologies社を買収した。これは固体/液体ロケットやスクラムジェットの生産コスト低減を狙ったもので、添加剤製造は「我々のソリューションに不可欠だ」とEvans氏は述べた。
以上は、Guy Norrisが Aviation Week & Space Technologyいた記事です。 Aviation Week & Space Technology は、豊富な経験と人脈を持った専門家により、最新トレンドや最適な状況判断、ポリシー・要求仕様・予算に関する充実した情報を継続的にお届けします。 Aviation Week & Space Technology をもっと知りたい場合、こちらをクリックして下さい。
After decades of promise the age of operational hypersonic vehicles is dawning, and concepts once considered the stuff of science fiction or the fanciful prediction of military planners are about to become reality.
The first generation of U.S. tactical boost-glide and air-breathing strike weapons are in development and will be fielded within a few years. But proponents say much more work still needs to be done before larger, next-generation designs can harness the advantages of flight speeds of Mach 5-plus for broader roles including strike and reconnaissance, high-speed civil and military transport and even multistage access to space.
Ranging from first order issues such as propulsion type selection and the complex coupled optimization of propulsion and airframes, designers must also contend with challenging changes in flow physics with varying Mach numbers. Added to the continuing quest for exotic materials with such high-temperature resistance that they have yet to be invented, the list of hurdles remains as daunting as ever.
Tests of rocket-boosted hypersonic glide vehicles and early air-breathing scramjet-powered demonstrators have, however, given today’s developers a solid foundation of the basic high-speed aerodynamics and propulsion requirements. “The good news is there is now zero doubt that a scramjet can be built and can produce net positive thrust,” says Mark Lewis, director of defense research and engineering for modernization at the U.S. Defense Department. “That’s all pretty remarkable. We have a keen understanding what the properties of the fuel should be and how to inject it effectively.”
But to go beyond rocket-boosted single-use gliders and missiles to fulfill the ultimate vision of developing reusable hypersonic aircraft will require a huge effort, says Lewis. “There’s a lot to do there in terms of the engine-airframe integration, particularly the propulsion transition,” he says. “We need a propulsion system that gets us all the way from Mach 0 all the way up to Mach 5 or 6 and then back down to Mach 0. That’s one we’re still working on. The big question is, should it be a combined cycle engine or multiple engines? Can I go direct from a turbine to a ramjet/scramjet, or do I need something intermediate?”
As the upper operating limit for turbine engines is around Mach 3 or 4, the focus for developers of air-breathing systems has been on a combination of high-speed turbojets, ramjets and scramjets—collectively dubbed the turbine-based combined cycle (TBCC)—to accelerate to Mach 5 and beyond. However, despite the pioneering success of the scramjet-powered NASA X-43A and U.S. Air Force-led X-51A vehicles, and tests in 2019 of a 13,000 lb.-thrust Northrop Grumman-built scramjet by the Air Force, there is no definitive consensus on the best path forward for integrated high-speed propulsion.
Part of the reason is that scramjets, while conceptually simple with no moving parts, are complex in terms of operational physics. The mechanical compression of jet engines is replaced by shock compression which, in the form of shockwaves entering the engine, must efficiently compress air within the inlet and isolator before turbulence and chemistry mix and react fuel and air in under 1 millisec.
Even by the performance standards achieved by the 50-in.-long combustor in Pratt & Whitney’s J58 turbojet of the 1960s, the fastest air breathing engine ever to seeoperational use, the task of the scramjet is orders of magnitude more difficult. The J58 traveled at 3,200 ft/sec at Mach 3.3 and 80,000 ft. in the Lockheed SR-71, while the X-43A’s 30-in.-long combustor traveled at around 9,600 ft./sec. as it flew at Mach 9.7 and 110,000 ft.
“The challenge is designing combustors with optimal overall geometries (area distribution and shape), injector and flameholder geometries, and the spatial distribution of fuel injectors, plus values of other fuel injection parameters,” says Kevin Bowcutt, the chief hypersonics scientist at Boeing who led the X-51A conceptual design. He thinks the hurdles, while significant, can be tackled using a multidisciplinary design optimization (MDO) approach that simultaneously combines aerodynamics, control, propulsion and structural considerations to produce a highly integrated solution.
“This design optimization process will be aided by fast, high-fidelity flow simulation [computational fluid dynamics] tools, good ground test facilities specializing in hypersonic engine testing, and sophisticated flow diagnostic instrumentation for test measurements,” adds Bowcutt, who has previously noted the X-51A was the first hypersonic vehicle to be refined using MDO.
However, despite decades of scramjet development work, competing propulsion concepts continue to emerge. So, are scramjets necessarily still the right answer? “I would say the jury’s still out,” says Lewis. “One of our challenges is to be open-minded enough to think maybe there are other ways to propel ourselves at high speed. I wouldn’t want to narrow down our technology options.”
Propulsion Options
A potential wide variety of alternative power cycles beyond the TBCC have also been studied that could speed a vehicle from a standing start to Mach 6-plus, most of which take advantage of the thermodynamic properties of liquid hydrogen as a working fluid for cooling and power. Examples include the ATREX expander air turbo-ramjet studied by JAXA, the Japan Aerospace Research Agency; the Russian-developed ATRDC deeply-cooled, air turbo rocket; the KLIN cycle thermally integrated deeply-cooled turbojet and liquid rocket engine; and the aspirating rocket engine.
Other air-breathing concepts showing promise include pressure-gain cycles such as rotating and pulse detonation engines and, potentially, even more exotic magnetohydrodynamic/magneto-plasmadynamic engines. Both cycles are being investigated for hypersonic application, the former for potential access to space as part of a pulse detonation rocket-based combined cycle with ejector ramjet, ramjet, scramjet and rocket modes.
Another alternative is the Reaction Engines SABRE (synergetic air-breathing rocket engine), which is in development at sites in the UK and U.S. Designed to power vehicles up to Mach 5.4 in air-breathing mode and all the way to orbital velocity in rocket mode, the engine’s precooler passed a critical milestone test in October when it operated successfully at temperature inlet conditions equivalent to Mach 5.
“The whole idea of a liquid air cycle engine and the deeply-cooled cycle is extremely promising,” says Lewis. “I don’t know if at the end, they’ll pan out, but they seem to have some significant advantages for certain classes of vehicles. I do worry that we [must] maintain a sufficiently diverse research portfolio and development portfolio that there is room for a full range of clever ideas.”
Airframe-Propulsion Integration
Air-breathers must navigate a relatively tight flight trajectory propulsion corridor as speed and altitude increase. Commencing with a takeover speed of around Mach 3, hydrocarbon-powered scramjets hit a fuel thermal limit around Mach 7.5, while hydrogen-powered vehicles reach a performance limit around Mach 14. In terms of altitude, most dual-mode scramjets are theoretically bounded by upper operability limits at a dynamic pressure of 500 psf—a level that runs from Mach 5 at 100,000-ft. to Mach 15 at 150,000 ft.
At lower altitudes, the denser atmosphere presents another barrier. Vehicle designers usually target hypersonic flight in the stratosphere to limit the dynamic pressure to below 2,000 psf. The line representing the resulting structural design limit extends from around 40,000 ft. at Mach 2.5 to around 110,000-ft. at Mach 14.
To add to these challenges, design considerations are also complicated by changing aerodynamic behavior with Mach number; finding the optimum wing size to suit varying flight modes; configuring the vehicle for a high propellant fraction; and defining the correct inlet and nozzle size, both of which vary extensively with Mach.
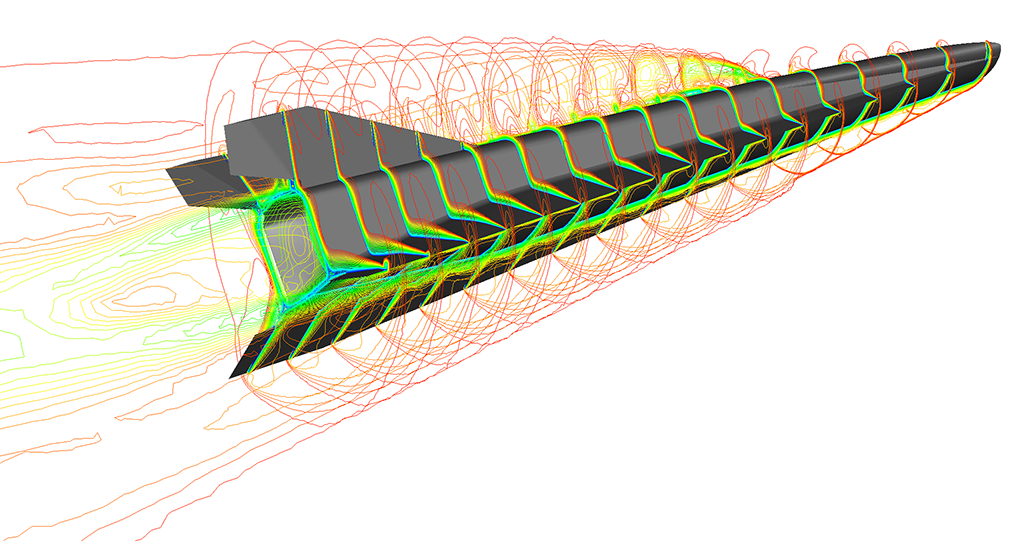
“We also know how to build good inlets,” says Lewis. “But there is still an ongoing question: What is the best inlet design? Under the general heading of ‘perfection is the enemy of good enough,’ we know how to build inlets that work. However, there are clearly areas for continued development, research and advancement.”
As achieving a high thrust-to-drag ratio is critical for efficient acceleration to Mach 5-plus, propulsion integration is vital and drives the need for increasing air capture with Mach number. Designers have discovered that overall air capture and engine thrust coefficient must be sufficiently high enough to give a thrust/drag ratio of greater than 2 at all speeds. But, at the same time, although inlet size and air capture must be relatively large for hypersonic performance, drag from inlets and nozzles must be reduced as the vehicle passes through the transonic region around Mach 1.
“It is very challenging to design inlets that accommodate a very large range of air mass capture (measured as capture area) and contraction ratio with high compression efficiency and flow stability, and low flow distortion and additive (including spillage) drag, across a large range of Mach number, altitude, and angle of attack” says Bowcutt.
“Over the years I’ve been involved in looking at conceptual design for a number of hypersonic vehicles where the final shape was dictated not by its hypersonic performance, but by the transonic performance,” Lewis recalls. “You can design hypersonic shapes that you know do great at Mach 5, 6, 7, 8, but they have no hope of punching through Mach 1. There’s a slight disconnect there. With the weapons applications we have an easier task because we are usually punching through Mach 1 with a rocket, for example. But this shows that low Mach number performance becomes critical.”
The extreme challenges of bringing together a closely coupled airframe and propulsion system are further magnified by the need to then minimize the weight, power and size requirements of the subsystems inside the vehicle. The need for a highly integrated flight architecture, made possible through MDO-type approaches, is “absolutely a key area,” says Lewis. “We knew 35 years ago that a hypersonic vehicle would have to be a fully integrated system, and today everything that we’ve learned has reinforced that,” he says.
Scaling and Flow Physics
Challenges also differ, depending on size. “Going to the bigger vehicles there’s another coupling that’s really important, in that structures can bend and flex,” says David Van Wie, head of the Air and Missile Defense Sector at Johns Hopkins Applied Physics Laboratory, Maryland. “You just can’t make it rigid enough,” he says. “Flight loads can couple back into the engine and the propulsion systems, and the degree of coupling gets more challenging as you go to larger scales.”
Smaller scale vehicles, though structurally stiffer, face challenges related to packaging and thermal management. “NASA studies long ago said long slender vehicles had much higher hypersonic lift-to-drag ratios than shorter, stubbier vehicles,” says Van Wie. “And when you go try to make something small, it can’t be long and slender, there’s just no internal volume. It ends up setting what is achievable from an aerodynamic efficiency from a lift-to-drag ratio.”
“There is a challenge there doing all the aerodynamics and propulsion integration with the internal packaging, Lewis adds. “You must make sure you have room for all the parts inside that you need such as sensors and systems for guidance, navigation and control.”
Another scaling issue, and one that goes to the heart of hypersonic vehicle design, concerns the behavior of the boundary layer, a thin layer near the surface that designers strive to keep laminar for as long as possible to minimize heating and thus reduce thermal protection requirements. At hypersonic speeds, the boundary layer tends to thicken and, in general, become more resistant to disturbances. But when it transitions to turbulent flow it can affect heating, drag and stability. Pressure rises across shockwaves can also interact with the boundary layer.
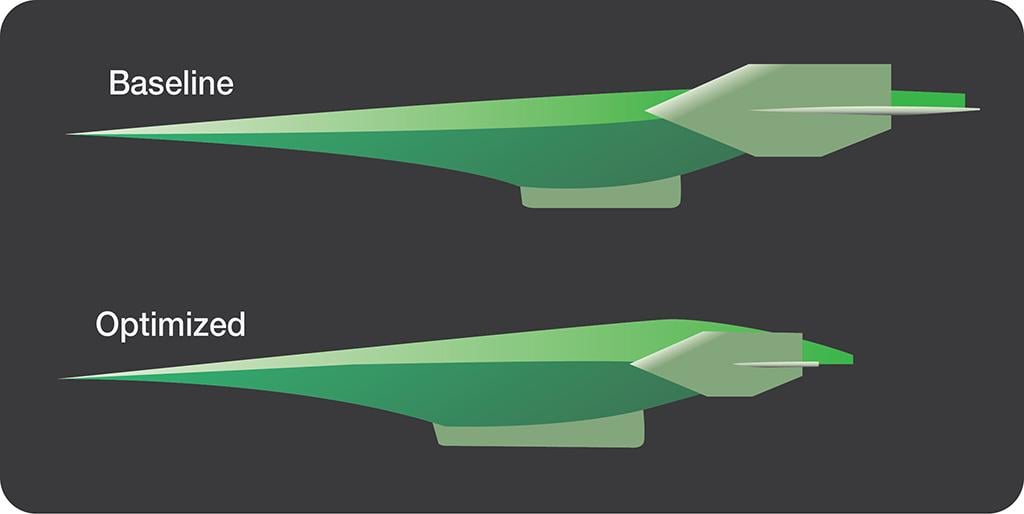
Although varying shock positions are in themselves not an issue, shock interactions with boundary layers produce friction drag and in some cases can magnify aerodynamic heating up to eight times higher than laminar flow. As shock locations change, so will interaction zones. “Therefore, areas of enhanced aeroheating will move around, requiring enhanced thermal protection or management over a greater area of the vehicle (increasing weight and cost),” explains Bowcutt. “Being able to accurately predict boundary layer transition is important. Developing and using means to delay boundary layer transition could also be important if they could be developed.”
Although advances in computational codes have recently enabled more accurate predictions of flow physics, further improvements in narrowing down boundary layer transition zones will help build robustness into hypersonic vehicle design, says Lewis. “We’ve learned to design around what we know we don’t know,” he says. “We realized we’re never going to know exactly when the boundary layer transitions from laminar to turbulent, so we have to design around that.”
Conversely, scramjet engine developers face the challenge of encouraging turbulent, rather than laminar, boundary layer conditions in inlets for better operability. “A turbulent flow is less susceptible to boundary layer separation where shocks interact with the boundary layer,” says Bowcutt.
Recalling lessons learned from working as a student on the National AeroSpace Plane (NASP) program of the 1980s, Lewis says a very senior lecturer warned that if the vehicle’s boundary layer was turbulent instead of laminar, then it would never fly. “Among the things I’ve learned is, if our vehicle designs are that sensitive, then they’re not going to fly and we need to be designing robust configurations,” he says. “That’s the hard part.” (See Aviation Week hypersonics timeline through 2017.)
Maturing hypersonic vehicles into operational systems remains a major hurdle, agrees Van Wie. “Improving our knowledge on how to actually build vehicles and get them to operate robustly in the environment is, I think, one of the bigger challenge areas right now,” he says. “You want to be able to fly it in a way where you don’t have to thread the needle and follow, for example, a given experimental flight profile. I want to fly it like any other aerospace vehicle.”
Interaction of the shockwave around the leading edges of the vehicle with the boundary layer is another challenge, particularly for slender-shaped boost-glide vehicles at higher altitudes and sharp-nosed reentry bodies. The degree to which flow interaction effects, or leading-edge viscous interaction, can occur is influenced by the Reynolds (Re) number, a nondimensional scaling metric that is the ratio of inertial forces to viscous forces. In the upper atmosphere with lower Re, the thickness of the boundary layer increases and interacts with the adjacent flow. At even the very sharp leading edges of a hypersonic vehicle, in very low Re, the growth of the boundary layer effectively changes the shape of the leading edge, making it seem blunter to the oncoming flow outside of the boundary layer.
The effect changes the air distribution at the leading edge, which in turn affects the behavior of the boundary layer and creates viscous interactions with potentially damaging impacts on lift/drag. Similar effects can cause trouble in other shock/boundary layer interactions such as inlets and on inlet ramps. “That’s a significant factor in the behavior of leading edges, but it introduces a scale,” says Lewis. “So, if I take a given shape that flies hypersonically with a certain performance, and I photographically reduce it. I could suddenly pick up entirely different physical behavior because of the way these interactions scale.”
Higher-Temperature Materials
Another significant challenge is in materials. Despite the seven decades of research that led to the development of thermal protection systems (TPS) and other heat-resistant treatments for spacecraft, ballistic weapons and supersonic aircraft, none of these material systems specifically meet the unique heating challenge of extended hypersonic flight through the atmosphere.
At sustained speeds of Mach 6 and above, the temperatures at the leading edges of hypersonic vehicles can run from 1,600-2,200C (2,900-4,000F), the upper range of which is more than 300C higher than the melting point of titanium and 600C hotter than the melting point of steel. The highest heat flux is focused, blowtorch-like, over a relatively small area, leading to high thermal shock and extreme temperature gradients. At such high temperatures, material properties can change during the flight, while oxidation and catalysis effects are also possible.
“It is an area where there’s a lot of opportunity for invention,” says Van Wie. “As the leading edges become sharper, they want to get hotter. That’s one of the main materials issues out there: How sharp do you want to go, and that fundamentally translates into the highest temperatures they can be operated at. The answers are influenced by whether it’s a reusable vehicle or a one-use weapon system. But this is an active area of research.”
“[Higher-temperature-resistant materials] would allow you to design vehicles with sharper leading edges, which would give you a higher lift-to-drag ratio for better performance. Or it would give more margin by allowing flight at higher speeds and altitudes,” says Van Wie. “It is a one of the challenge areas where there’s a lot of room for improvement.”
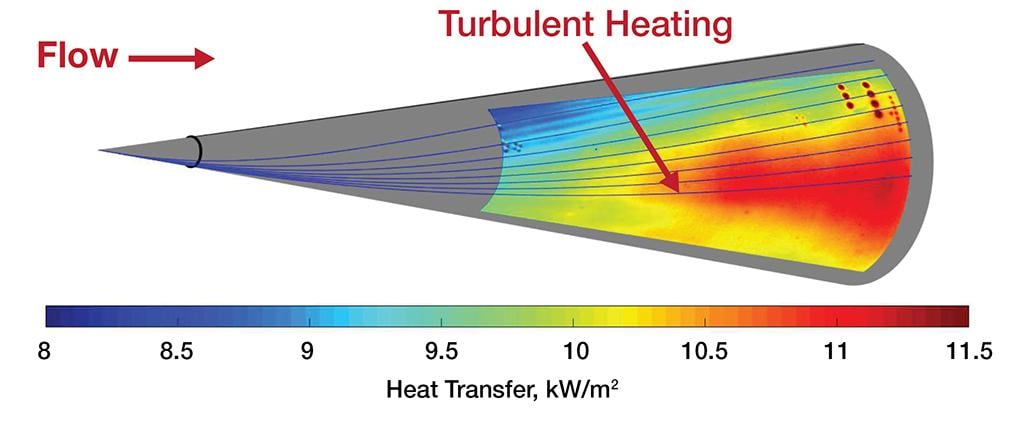
Another significant, related, hurdle is the changing thermal environment with varying vehicle size. “People still don’t understand how the material challenges scale with size,” says Lewis. “It’s another avenue that requires continued investment and development. For example, if I take to make a given hypersonic configuration smaller by just photographically reducing it, then I wind up with much sharper leading edges. And then those get much hotter. So, I end up with this trade-off going on for blunt leading edges that create more drag and have less aerodynamic performance.
“So that’s a pretty active area, especially as we’re talking about delivering hypersonics at scale. And for doing it in large numbers, we realized we had to be very careful extrapolating that if something works on a certain scale, it doesn’t mean that we can take that same configuration and apply it across the range of systems that we’re looking to develop,” he adds.
As a vehicle passes through the atmosphere at Mach 5-plus, the air itself can change. “Your temperatures become such that the chemical composition of the air flowing around the vehicle begins to change,” says Lewis. “You start to disassociate oxygen. Then at even higher temperatures eventually you can start disassociating nitrogen. This chemical dissociation affects the behavior of the flow over the vehicle.” The dissociation of diatomic oxygen into oxygen radicals takes place at temperatures around 1,700C, while that of nitrogen is at around 3,700C.
Disassociation, plasma formation at higher Mach numbers and aeroheating effects pose a major challenge for communicating with hypersonic vehicles, as well as for guidance, navigation and control (GNC). “It makes the hypersonic calculation somewhat challenging. Where do you put your sensor, and how do you put in an aperture or window of some sort that you can blend with the rest of the vehicle?” asks Lewis.
Research and development into tackling the GNC hurdles is active across the hypersonic enterprise, with current study work ranging from new sensors and avionics for missiles and transatmospheric vehicles to advanced designs for semiautonomous/autonomous missile guidance systems and development of new guidance laws for high-speed vehicle trajectory optimization. Other areas of study include the application of data fusion for target discrimination and tracking algorithms, as well as development of new adaptive control algorithms and novel actuation systems.
Materials represents the “first and foremost” challenge to successful hypersonic vehicle development said David Hunn, director of technology at Lockheed Martin Missiles and Fire Control. “I’m having to adjust my trajectory and performance based on the limitations of materials systems that are available now.” Hunn was speaking at a Royal Aeronautical Society hypersonics conference in London in November.
“We are in regimes that have rarely been explored in the past. So how do we handle that?” he asked. The options range from internally cooled structures with circulating coolant to insulated structures with passive, ablative or semiactive TPS. But overall, the radiation cooled/hot structure approach is preferred. “[It] is simple, there are no moving parts such as pumps, and it is predictable and stable,” he said. “Arguably it also offers the minimum space, weight and power.”
Coating Challenges
With metals incapable of withstanding the required heat flux, material choices are currently focused mainly on carbon-fiber and carbon-matrix composites as well as ceramic composites.
“We have a hope here that there are material solutions out there but—and there is always a but—we have to understand that these carbon-based materials oxidize,” said Hunn. “So in the air I have to worry about chemical catalytic reactions breaking the air down into its base elements and recombining that with the airframe—and that would be an endothermic reaction adding more heat to it. I also have to worry about heat emissivity. If it is too low it gets really hot. Fundamentally, no matter what the airframe materials, the chances are we will have to develop protective coatings to work in these atmospheres.”
Work on new coatings for temperatures in excess of 1,700C are focused on novel ceramic materials such as tantalum and hafnium carbide, and zirconium and hafnium borides. “Some are ceramic-based materials, which are pretty good oxygen barriers, so there’s a lot of research looking at some of these novel coatings on some of these advanced composite materials as near-term solutions for current hypersonic airframes,” said Hunn.
However, to advance hypersonic vehicle development to the next level, he said, “The answer really is that we are going to have to sidestep Mother Nature and invent our own materials.” Work at a fundamental level is underway at universities and research labs that is focused on using computational power and a road map from the periodic table to “come up with some novel new materials that have the characteristics needed to roll into hypersonics,” he said.
Reviews of Chinese and Russian research work indicates “that they are really advancing the state of the art in designing new materials and tailoring them for the hypersonic environment,” said Hunn. Scientists from Moscow’s National University of Science and Technology, for example, recently revealed tests of a new ceramic compound they claim will withstand the typical 2,000C thermal loads of a sharp leading edge or nosecone of a hypersonic vehicle. The melting point of the new material, called hafnium carbonitride, is not yet known but has already been demonstrated to be beyond 4,000C, say the scientists, who add that further tests are planned at hypersonic conditions.
The corollary of the external heating issue creates an engineering and design challenge for the densely packed interiors of hypersonic vehicles—both gliders and powered. “You have an outside surface that wants to be hot and you have electronic systems inside that you can’t allow to get hot,” says Van Wie.
The problem is compounded by the insulation built into the vehicle to prevent heat transfer from the exterior. “So, what do I do with the internally generated heat flux?” asks Van Wie. “There’s nothing magical about that problem—it’s just an engineering system challenge. There’s a whole variety of different ways of attacking it, but you have got to take a look at it from a system perspective and what makes sense for a particular vehicle type and a particular mission.”
Readying the Ramp-Up
A final and more urgent challenge, particularly for the first-generation U.S. weapon systems developers, is establishing the industrial base to support the planned production ramp-up. Earlier this year the U.S. Defense Department’s acquisition leadership established a “war room” in the Pentagon to assess the areas of strength and vulnerability in the industrial supply chain.
Propulsion specialist Aerojet Rocketdyne, which provided the scramjet for the X-51A, is actively engaged on a series of offensive and defensive hypersonic efforts, ranging from DARPA’s Glide Breaker and Operational Fires programs to the Advanced Full Range Engine TBCC propulsion system and the hypersonic air-breathing weapon concept (HAWC) with Lockheed Martin.
“We’re focused on executing the programs we’ve got and then having the capability and capacity to do what’s needed when the time comes,” says Tyler Evans, senior vice president of Aerojet Rocketdyne’s defense business. “The X-51 was a propulsion demonstrator that demonstrated that we could tame the science of supersonic combustion, and it did that. And so, 10 years later, we’re focused on making scramjets practical, making them repeatable, making them affordable.”
As part of the readiness ramp-up the company also acquired Florida-based additive manufacturing specialists 3D Material Technologies, in a move designed to support lower production costs across its range of solid and liquid rockets as well as scramjets. Additive manufacturing is “integral to our solution,” says Evans.