
If you blow a cabin at mid-ocean, can you make your alternate?
Oh, we humans, never content to remain on the earth that holds us down with its gravity and sustains us with oxygen-laden atmosphere to breathe.
No, as soon as we figured out how to ascend into that atmosphere, cheating gravity with hydrogen-filled balloons or artificial wings, we were compelled to claw our way ever higher into the thin, cold air of the stratosphere. But alas, fragile mammals that we are, we could not survive for long – let alone maintain consciousness to control our fabricated aerial conveyances. So we learned to take containers of our precious gravity-thickened atmosphere aloft with us to inhale through rubber hoses or to encase our bodies in suits and helmets pumped full of that life-fortifying gas.
Auto Emergency Descents: Watch Out Below
In the early 1930s, record-setting aviator Wiley Post and Russell Colley of B.F. Goodrich Co. developed some of the first pressure suits — looking more like deep-sea diving rigs — for long-distance, high-altitude flights. Wearing one of these in 1934, Post achieved an altitude of 40,000 ft. over Chicago, piloting his famous Lockheed Vega Winnie Mae. Swaddled in his pressure suit on a subsequent flight, he climbed the Vega to 50,000 ft. and inadvertently discovered the jet stream.
Later in the decade, military aircraft were introduced that were powered by supercharged engines capable of routine flight into the mid-30,000s, where crewmembers breathed bottled oxygen and endured sub-zero temperatures wearing insulated flight suits. But for commercial transportation at high altitudes, to avoid weather and attain higher speeds, oxygen masks or pressure suits would be impractical for passengers, so an appropriate, comfortable cabin environment would be needed.
Perfecting the Pressurized Cabin
Boeing took on this challenge later in the decade and, with a pressurized cabin mated to the flying surfaces and engines of its B-17 Flying Fortress heavy bomber, produced the Model 307 Stratoliner, the world’s first pressurized airliner. During World War II, the small number of 307s that had been produced for Pan American World Airways and Trans World Airlines was drafted into the Army Air Force as C-75 transports (sans the pressurization equipment to save weight). Meanwhile, Boeing lofted what it had learned in the program into the B-29 Superfortress, the world’s first pressurized bomber, a quantum leap forward in aircraft design.
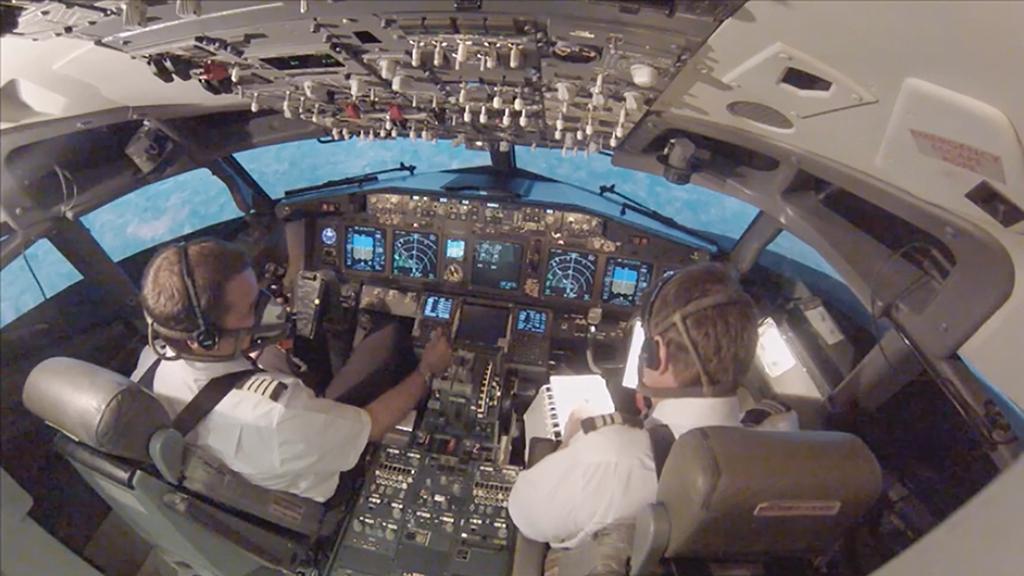
At war’s end, Boeing once again leveraged a military design into a commercial airliner, using the B-29 airframe to create the double-decked KC-97 military transport/tanker and Model 377 Stratocruiser civil counterpart. Douglas and Lockheed quickly followed with, respectively, the DC-6 and -7 and Constellation pressurized piston-powered airliners, setting the stage for the emergence of the first-generation jetliners.
The British led the way with the de Havilland Comet, an aesthetic tour de force but initially plagued with a design flaw that led to metal fatigue in the upper fuselage from repeated pressurization and decompression cycles each time the plane flew. However, that aircraft initially had a design flaw causing metal fatigue in the upper fuselage from repeated pressurization and decompression cycles, which resulted in catastrophic decompression and inflight breakup of two Comets and loss of all on board. (A third crash occurred when a Comet was overstressed in violent weather and also broke apart in the air.) The ensuing investigations led to a fleet grounding and redesign of the type. Significantly, the effort also resulted in a major revision of the British Civil Aviation Authority certification rules, recasting the CAA as the most stringent aircraft approval agency in the world.
Meanwhile, Boeing spawned the iconic Model 707 from the KC-135 jet transport/tanker it had developed for the U.S. Air Force. And Douglas and Convair followed, certificating their contributions to the civil jet age, respectively, the DC-8 and 880/990. These transports were designed to cruise at altitudes up to 41,000 ft. for best efficiency of their turbojet engines, requiring robust and reliable cabin pressurization systems with differentials as high as 8.5 psi. In the early 1960s, the Lockheed JetStar, North American Sabreliner, Learjet 20/25, Beech King Air and Grumman Gulfstream debuted, bringing turbine-powered, high-altitude speed and pressurized comfort to business aviation.
Without cabin pressurization or supplemental oxygen to breathe at such altitudes, flight crews and passengers would quickly be overcome by hypoxia — oxygen starvation — followed rapidly by unconsciousness. Death would occur soon afterward. It is a tribute to designers and manufacturers of pressurization equipment (or “packs,” when installed in airframes) that humans can routinely venture into the stratosphere, traveling in comfort at average 7,000-ft. cabin altitudes, while the outside air is unbreathable and ambient temperatures hover in the -60s F (-50s C).
Get Down — Fast!
While sudden cabin depressurizations in flight are rare, they can occur, and all pilots of aircraft built to climb and cruise above 12,500 ft. are trained to cope with them. The standard procedure for an unexpected loss of pressurization is an immediate and rapid descent to a lower altitude where aircraft occupants can breathe without emergency or supplemental oxygen, generally around 15,000 ft. However, some operators of turbine-powered equipment may choose a recovery altitude as high as 25,000 ft. for more favorable fuel burn on the way to an alternate.
In two-crew cockpits, one pilot will execute the descent while the other communicates with air traffic control to clear any aircraft flying below away from the descent area. With loss of cabin pressure below a predetermined value, emergency oxygen masks should drop from ceiling compartments above every seat in the cabin. Note that loss of cabin pressure and donning of emergency masks should be part of passenger briefings.

On March 9 of this year, a Southwest Airlines Boeing 737-300 on a fight from Las Vegas to Boise, Idaho, experienced a gradual loss of cabin pressure while cruising at 39,000 ft. Noting the pressure drop on cockpit instrumentation, the crew initiated a 6-min. descent to 22,000 ft. and continued uneventfully to the destination. Because the pressure loss was gradual and not catastrophic, the emergency oxygen masks did not deploy. A post-flight inspection of the aircraft revealed a 12-in. crack in the crown of the fuselage just aft of the cockpit. Southwest claimed the airframe had been inspected for skin cracks during the previous FAA-required 1,500-hr. airframe inspection interval. (Apparently, the fuselage crown — which experiences heavy slipstream pressure in flight — is a somewhat common area for fatigue cracks.)
The carrier, whose all-737 fleet logs high cycles (as many as five takeoffs and landings a day), is no stranger to cabin depressurization incidents. It experienced three rapid depressurizations in a 30-day period in 2018. The first occurred on April 17 when the left engine of a 737-700 threw a fan blade into the fuselage, killing a passenger in a window seat and depressurizing the cabin; the aircraft executed an emergency descent and landed at Philadelphia. On May 2, a 737 en route to Newark from Chicago was forced to make an unscheduled landing at Cleveland after the outer pane of a window cracked. And on May 12, a flight from Denver to Dallas experienced what the airline termed “a pressurization issue” with emergency oxygen masks dropping from ceiling compartments 30 min. before the flight landed at Dallas; some passengers experienced pain in their ears, and the crew radioed ahead for medical personnel to meet the flight.
In 2009, a Southwest 737 cruising at 35,000 ft. over West Virginia lost cabin pressure when the bond between two aluminum skin plates separated on the top of the fuselage; an emergency descent and unscheduled landing ensued. And in April 2011, an explosive decompression blew a 5-ft.-long hole in the roof of one of the airline’s 737s flying from Phoenix to Sacramento, California. Passengers reported a loud bang and being able to see the sky through the oblong opening. Oxygen masks dropped as pilots initiated a rapid, controlled descent for an emergency landing at an Arizona military base. Fatigue cracks in the plane’s skin were blamed for the failure.
Two of the most spectacular and horrifying airline depressurizations occurred in the late 1980s, both involving older Boeing airframes.
On April 28, 1988, Aloha Airlines Flight 243, a 737-200, was en route from Hilo to Honolulu at 24,000 ft. when an explosive decompression caused an 18.5-ft. section of the top half of the fuselage from behind the cockpit to just forward of the wing to depart the aircraft. A flight attendant standing in the aisle was sucked from the aircraft. Looking over their shoulders to where the cockpit door had been, the flight crew could see blue sky. Controls were responsive, and the pilots immediately began a descent, steering the stricken aircraft to the nearest alternate, Kahului Airport on the island of Maui, performing a successful landing 13 min. after the fatal incident.
Emergency evacuation slides were deployed and the passengers and crew quickly evacuated the aircraft. Of the 94 survivors on the airplane, 65 were injured, eight of them seriously. The decompression occurred over open ocean, and the ill-fated flight attendant’s body was never found; she had served for 37 years with Aloha.
The 737-297 had been delivered to Aloha off Boeing's Renton, Washington, production line in 1969 and had logged 35,496 hr. However, due to the short-segment, high-cycle nature of Aloha’s intra-island service, the 737 had experienced 89,680 cycles at the time of the decompression, more than twice the number of flights for which the aircraft was designed. It was considered to be irreparable and was dismantled on site.
The NTSB determined the cause of the explosive decompression was metal fatigue exacerbated by corrosion in a bonded lap joint in the upper fuselage skin. It is notable that the entire 19-year operational life of the aircraft had occurred in a high salt and humidity marine environment, rife for corrosion. The Safety Board also cited the fabrication process Boeing was employing at the time to bond 737 upper-fuselage sections, leading to a revision of the process whereby a doubler was applied over the lap joint that had failed in the subject aircraft. Aloha management was also cited for failure to properly supervise its maintenance department and correctly carry out required airframe inspections. The FAA also caught some blame for not requiring Airworthiness Directive 87-21-08, which directed inspection of 737 lap joints as per a Boeing Service Bulletin and a complete terminating action “after discovery of early production difficulties in the 737 cold-bond lap joint, which resulted in low bond durability, corrosion and premature fatigue cracking.”
The second incident occurred 11 months later and involved a United Airlines Boeing 747-122 on a flight from Honolulu to Sydney with 337 passengers and 18 crewmembers aboard. As the aircraft was climbing out of PHNL and passing through 22,000 ft., the forward baggage door overcame its latching system and blew out so explosively that it slammed back against the fuselage on its hinges, opening a gaping hole and decompressing the cabin. The cabin floor caved in from the pressure differential, and 10 seats occupied by eight passengers were ejected from the hole; a ninth was sucked out from a seat still in the cabin. Engines three and four on the right wing suffered debris damage and eventually both had to be shut down. A flight attendant was nearly sucked out, too, but saved herself by hanging onto the aircraft’s upper deck stairs until she could be pulled to safety.
The cockpit crew, assuming that a bomb had gone off in the hold, immediately began a descending left turn back to Honolulu. Because the right-wing flaps had been damaged and could only partially be deployed, the crew calculated a landing speed of between 190 and 200 kt. The landing was successful, and Capt. David Cronin was able to bring the big Boeing to a stop on the runway. All of the victims pulled out of the aircraft were lost at sea.
The 747 was manufactured in 1970, and at the time of the cargo door blow-out had accumulated 58,814 hr. and 15,028 cycles. The cargo door was eventually recovered by a robot submarine under 14,100 ft. of Pacific Ocean. An extensive two-part NTSB investigation (the second part was a reopening of the original investigation after the cargo door had been recovered) determined that the cause of the accident was “the sudden opening of the cargo door, which was attributed to improper wiring and deficiencies in the door’s design. It appeared in this case that a short circuit caused an uncommanded rotation of the latch cams, which forced the weak locking sectors to distort and allow the rotation, thus enabling the pressure differential and aerodynamic forces to blow the door off the fuselage; ripping away the hinge fixing structure, the cabin floor and the side fuselage skin; and causing the decompression.” The Safety Board recommended that locking systems for outward-opening cargo doors on this variant of the 747 be replaced and redesigned.
Notable Business Jet Failure
Business jets and pressurized turboprops have cabin pressurization lapses, but rarely. Probably the most tragic and arresting was the 1999 case involving a Learjet 35 chartered by professional golfer Payne Stewart and three colleagues for a flight from Orlando to Dallas. Climbing out on a northwesterly course with 4 hr. of fuel on board and a clearance to FL 390, Jacksonville Center lost radio contact with the aircraft as it passed through 23,000 ft. Then the Learjet failed to make a planned turn toward Dallas, climbing through its assigned altitude and ultimately reaching 48,900 ft. on its original course.
When repeated attempts to contact the flight crew went unacknowledged, controllers requested an Air Force F-16 pilot out of Eglin AFB who was flying nearby to intercept the Learjet and make a visual inspection. After also failing to receive a radioed response, the fighter pilot closed in on the Learjet and reported no visual anomalies with the aircraft; both engines were running, and the rotating beacon was activated. However, moving closer, the pilot noted that, while the cabin windows were dark, most of the cockpit panes were frosted over and he could see no movement inside the aircraft. Then he had to break away because of low fuel.
As the Learjet continued north, two more intercepts by Air Guard F-16s from different states were carried out with no reported changes to the business jet or its behavior. By then, it was assumed that the Learjet’s crew, and probably its passengers, had become incapacitated, probably from a cabin depressurization, that the aircraft was operating on autopilot, and that it would probably fly until its fuel ran out — or it was shot down so it wouldn’t threaten a populated area like a city.
(During the aftermath of the event, Pentagon sources strongly denied that shooting down the errant Learjet was ever an option. However, the prime minister of Canada did authorize the Royal Canadian Air Force to destroy the Lear if it entered Canadian airspace, as its unwavering course would have taken it directly to Winnipeg.)
In the end, the Lear exhausted its fuel over South Dakota. When the autopilot began pulling the aircraft’s nose up, attempting to maintain altitude, the stick shaker, sensing an incipient stall, disconnected it, sending the Learjet out of control and nearly reaching supersonic speed as it spiraled down into an open field. The two pilots and four passengers aboard either succumbed early in the flight or died in the crash. The Lear had traversed 1,500 sm in just under 4 hr.
The subsequent NTSB investigation assumed that the Lear 35 had suffered a cabin depressurization and that occupants had died from hypoxia. But a definitive cause of the decompression was elusive. Impacting the ground at a steep angle, little was left of the aircraft that could determine exactly what had caused the cabin depressurization or the nature of it — a rapid decompression or a very subtle leak-out of the pressure vessel. As there was no evidence of a breach, based on the visual inspections of the F-16 pilots, logic tended to support the latter possibility, a gradual loss of pressure and stabilization of the cabin altitude to that outside the airplane.
Why No Supplemental OX
A blown seal somewhere or a faulty flow control valve could have caused a subtle drop-off. Considerable testing by the NTSB supported the premise that a closed flow control valve could cause complete depressurization over a period of several minutes. Then, consider the Learjet 35’s location of the cabin pressure gauge and associated controls at the bottom left-hand corner of the first officer’s panel — i.e., hidden behind an average human’s knee and not within the usual scan pattern. The crew could have missed the gauge’s unwinding until the falling oxygen levels had impaired their cognitive abilities to the point where they couldn’t respond to the cabin altitude alert or don their supplemental oxygen masks.
Tests showed it would take only a few minutes for cognitive abilities to be compromised. From the NTSB accident report: “If there had been a breach in the fuselage (even a small one that could not be visually detected by the inflight observers) or a seal failure, the cabin could have depressurized gradually, rapidly, or even explosively. Research has shown that a period of as little as 8 sec. without supplemental oxygen following rapid depressurization to about 30,000 ft. may cause a drop in oxygen saturation that can significantly impair cognitive functioning and increase the amount of time required to complete complex tasks.”
Finally, it is noteworthy that the Learjet 35’s operator had documented several instances of maintenance on the aircraft’s pressurization system leading up to the accident. The NTSB, however, was never able to substantially verify that any act of maintenance or specific component in the pressurization system was responsible for the loss of cabin pressure, i.e., that “nothing stemmed from a common problem.” Its stated probable cause of the mishap was “incapacitation of the flight crewmembers as a result of their failure to receive supplemental oxygen following a loss of cabin pressurization, for undetermined reasons.”
Another depressurization incident involving a Learjet 35 about a decade earlier had a much happier outcome for Joe Hotkewicz, who currently captains an intercontinental business jet for a corporate flight department. His tale began with a takeoff from a New Jersey airport in the Learjet 35, filed for Charlotte, North Carolina. “We were climbing to the southeast for our assigned cruising altitude of 39,000 ft. when I felt something in my ears — a clicking — as we passed through FL 330.”
Simultaneously, Hotkewicz and his copilot noted the cabin altitude was rising at 2,000 fpm and knew immediately what was happening. “In rapid succession, we donned our oxygen masks, radioed ATC, and I initiated a descent to 10,000 ft., which took about 2.5 min.,” he said. “This happened about halfway through the trip, and we continued on to Charlotte for a routine landing.”
Hotkewicz had six passengers aboard the Lear that day. “We were lucky. This was my first flight in a Learjet. I had just completed type training, and our chief pilot was riding the jump seat as check airman, and he handled the people in the back. The emergency oxygen masks correctly deployed in the cabin.”
Later, Hotkewicz learned the depressurization had been caused when ducting for the bleed air from the engines had come apart, and the hot air was blowing into the “hellhole,” the aft services compartment between the engines. Further, the point where the break occurred was in contact with a wiring bundle, and the hot air was melting the insulation on the wires.
A Happier Ending . . .
Another business jet depressurization, this one affecting a Dassault Falcon 2000 in 2018, was the progeny of a collection of multiple incidents that began with a ferry of the airplane from a southern city to Teterboro, New Jersey. Our narrator, who asked for anonymity, accepted the airplane for a trip to Europe with planned multiple stops. “On the ferry,” he said, “the crew had a pressurization problem that they isolated to the auto controller.” They transferred the system to manual mode, and that kept the cabin where it should have been.
“Then I picked the trip up,” the narrator continued, “and we headed for Biggin Hill outside of London, and everything went fine. We made several stops in Europe without incident, and then, going from Avion to Bordeaux with three passengers aboard, the same problem with the pressurization system auto controller came up again during the climb out. We went through the checklist, isolated the auto controller, and adjusted the cabin with the manual controller. We landed at Bordeaux — site of the Falcon factory — but there was no one there to work on it, so we then headed to our next destination, Oslo, and everything worked great including the auto controller.”
The final destination of the Falcon 2000 was back in the U.S. in Colorado, “so just to be safe and ensure we would have alternates we could make if we had to do a rapid descent to a low altitude,” the narrator explained, “we took a northerly route over Iceland, Greenland and Frobisher Bay. It was a great circle route that was better anyway, and presented lots of alternates in case we would have needed any.” (Note the selection of the route for access to good alternates in the event of a depressurization.)
Still another pilot BCA interviewed experienced a catastrophic depressurization on a ferry flight in the Southwest U.S. during the last century when a baggage door blew out on a first-generation business jet at cruising altitude. “The first thing I remember,” he said, “was how cold it got in the airplane.” The crew and a third pilot riding in the jump seat immediately donned emergency (supplemental) oxygen masks, alerted ATC, descended to an altitude with breathable air and then executed a safe emergency landing.
Every cockpit crew of an aircraft that operates into the stratospheric altitudes must take the possibility of a cabin depressurization into consideration in their flight planning, i.e., where will you go if you have to descend into lower altitudes with consequent fuel consumption issues. This consideration is paramount in long-range flights across remote regions of the planet.
Can You Make the Alternate?
Here is the premise of this discussion: How do you plan “suitable” alternates to accommodate loss of cabin pressure on very-long-range flights over remote areas, such as the North Pacific? Loss of cabin pressure is insidious: For the survival of all on board, you have no choice but to go down — and as we’ve seen, the faster the better. The drill: Don supplemental oxygen masks, 45-deg. turn off course, inform ATC, and descend like a stone to get down to an altitude where you can breathe without the aid of bottled oxygen.
“It is easy to see where vast expanses of ocean like the Pacific can be problematic in alternate selection,” Guy Gribble, president, International Flight Resources, a training consultancy, observed. “Then, too, there are continental regions that can be thought of as ‘dry oceans,’ — for example, Western China, Eastern Russia, the Amazon Basin, the Australian Outback and the trans-polar routes that were opened up only a couple decades ago.” These unique regional conditions must be evaluated for hazards demanding conscious risk-mitigation measures for acceptable remote alternate airport selection. “It goes without saying that over open ocean like the South Atlantic and Pacific,” Gribble pointed out, provocatively, “your aircraft simply has to have the range to get to a runway in the event of a contingency.”
Added Mitch Launius, of 30 West International Procedures Training, “The Pacific is challenging, but so is northern Russia, and particularly so are polar operations. There are places to land but the options are poor. People with medical problems can die on the ground because medical facilities are so far away.” All this has to be considered in the selection of alternates.
So, when it comes to alternates and long-range flights, the more planning the better, Gribble, a retired American Airlines widebody captain, insists. “The PIC must be involved early in the process by engaging the flight planning service’s experience and forecasting ability. Operating pilots need to set the priorities and define the acceptable conditions for alternate selection from the start. This can be refined closer to departure time. This is not as simple as having an airfield nominated by a handling service and an equal time point [ETP] calculated for you.”
Launius agreed. “Equal time points are what this is all about. Planning should be done under the advisement of the crew — it’s only a math problem for the flight planning agencies. It should involve more than just the minimums that are required to make this work. Select an altitude for what would be safe for your passengers, plan diversions to fields with appropriate medical capabilities. It’s our business to know what the best choices are and not just accept what the planners give you.”
Questions on the runway condition, length and airport services should play a role when selecting an alternate. What is the runway condition? Is it long enough? What are the surface conditions? (“The really important piece,” to Gribble.) Is there a displaced threshold? Is runway maintenance in progress? “Be aware that a Landing Runway Condition Assessment [LRCA] prior to approach, originally a commercial ops requirement, is now a requirement under Part 91, as per SAFO 19001,” Gribble reminded operators. “EASA has the same thing via ICAO, effective November 2020. If you slide off the runway some place, the regulating authorities will want documentation that you had done the assessment [e.g., field condition reports via the FICOM NOTAMs — see AC 25-32 for details].”
Know Your Runway
And once you get to that runway, you have to know whether you can you stop the airplane. “Consider, for example,” Gribble pointed out, “that in the Amazon Basin, some runways can be covered with a green fungus activated after the frequent rainfalls that characterize this tropical region. This makes for a temporarily super-slick runway surface.” Another consideration: Once safely stopped on the runway, what is your next move? Is there a parallel taxiway to the ramp or is a back taxi on the runway required? On the ramp, is there parking available and is the surface sufficiently “hard,” i.e., what is the Pavement Classification Number (PCN)? Is it enough to support your aircraft weight and wheel configuration, the Aircraft Classification Number (ACN)?
There may not be ramp service, fuel available or a hotel on or near the field. Most en route diverts are driven by medical concerns for the crew or passengers. So what kind of medical services are available on or near the airfield? Consider the fact that first aid or emergency medical service (EMS) at a commercially served airport is only on duty for the commercial flight service. And once that last commercial flight has landed safely, those services are closed, and the personnel manning them go home. In most austere regions, the availability of a fully staffed trauma center will be non-existent or a long drive or ambulance ride away in the closest city. Now, compare this to a hypothetical onboard medical response you may have and the transit time to a full-service trauma center at a different alternate airport option.
“It is equally important to consider cultural issues,” Gribble reminded operators. “Many remote regions are especially sensitive to religious and political orientations. Ongoing armed conflicts can impede expedited travel to a safe harbor of an en route divert airfield. These issues rarely offer any latitude for exceptions and are ever-changing with the local political system. This makes for a dynamic and ongoing security evaluation across planning and en route phases of the trip.”
Consider the Descent
The emergency descent demands consideration, also. “The underlying issues concerning rapid emergency descents are oxygen, or the lack of it, and terrain, too much of it,” Gribble cautioned. “While a reasonably healthy person may be able to endure a cabin depressurization and the subsequent rapid descent, the experience may not be the same for someone who is ‘pneumatically challenged’ with a condition like COPD, asthma or the effects of heavy smoking.” Other factors can include obesity, advanced age and lack of fitness.
According to Launius, “Depressurization, if it’s bad, can lead to situations that require medical attention — anything above 15,000 ft. exasperates that, and some operators use 25,000 ft. as their descent altitude for better fuel burn.
“Medical problems can appear in more ways than you can imagine,” he continued, “gastronomic, dental, anxiety, the bends. Most people don’t know the effects of flying a non-pressurized airplane at those lower altitudes. I’ve seen companies that plan for depressurization ETPs at 25,000 ft. for extended periods of time in an effort to meet fuel requirements.”
Scuba diving and recent blood donations exacerbate the physiological problems of cabin depressurization. A 24-hr. waiting period is generally accepted as the limitation for scuba diving; for blood donations, it’s up to three days. Of course, this will vary with individual health and fitness conditions. Following a scuba dive without at least a 24-hr. pause, in the event of a depressurization incident and subsequent rapid descent, there is a significant risk of encountering the bends, a condition known to divers where the dissolved nitrogen in the blood begins bubbling out of the circulatory system. And at the higher altitudes that some airplanes can fly today, without cabin pressurization, the diver’s blood can actually boil.
Exactly how you conduct the descent depends on the airspace in which you are flying. Take, for example, operating in an organized track system, such as the North Atlantic Track System (NATS) or Pacific Organized Track System (PACOTS). This is high-density airspace, and depending on your assigned flight level, there will generally be aircraft flying below you at 1,000-ft. vertical separations and to either side (if you are on an inside track), now at reduced lateral separation.
When lateral separation was 60 nm (or 1 deg.), the procedure for initiating a rapid descent was to turn either left or right from the assigned track 45 deg. and then begin the descent while informing the relevant air traffic manager of your intentions. But now that lateral separation has been halved to 30 nm (0.5 deg.), the cut from the assigned heading has been correspondingly reduced to 30 deg. Note that this does not leave a lot of room for maneuvering. Launius points out that “if a track has a ‘slope’ [i.e., angled in relation to the meridians of longitude], separation can be as close as 19 nm in some instances!”
The tracks are stacked as high as FL 410, but many business jets are certificated to fly well above that, and operators often elect to overfly the tracks, cutting across them at an angle — or will even “shadow” individual tracks. The lesson here is to always know what is below you, so make sure your planning chart shows the track array for the 12-hr. period in which you are operating and that you know your position relative to the tracks. This way, if you have to go down in a hurry, you’ll be able to make the descent between the tracks.
Terrain is a constant threat in executing a rapid descent to breathable air. “This requires pilots to be familiar with the definitions of MOCAs, MORAs and MEAs and their location relative to the aircraft’s position,” Gribble said. “How do I get to a safe altitude along this route to an alternate? This is as basic a requirement as looking for forced landing spots during primary flight training.”
A direct route from your present location to a diversion airport is not always possible due to terrain limitations. In such cases, a series of intermediate waypoints needs to be included in flight planning to delineate a suggested safe routing from a planned position to a diversion airport. “Keep in mind that it does little to plan a perfect terrain avoidance model at a higher-than-normal emergency descent altitude only to run out of supplemental oxygen for the passengers and possibly the crew part way to the alternate,” Gribble chided. “The same can be said of fuel consumption.”
Extended Range Ops and Alternates
Business aviation pilots operating under FAR Part 91 could learn a lot from the commercial world’s ETOPS, or Extended-Range Twin-Engine Operational Performance Standards. “There is a direct correlation with overwater and remote continental alternate selection for all operators, commercial or private,” Gribble asserted. “In either case, the concept of ‘suitable’ versus ‘adequate’ airports applies. An adequate airport is one that has a runway; a suitable airport is one where you can approach, land and stop and which has services available. An adequate airport may not have approach and runway lights or crash-fire rescue, but a suitable one would have these items. Suitable airports also have passenger facilities with enough room and temperature control to accommodate everyone on your aircraft.” In some cases, a passenger recovery plan needs to be planned and supported before operating with a particular airport listed as an alternate.
Another ETOPS concept applicable here is the “validity period,” the time window during which a designated alternate should be evaluated for landing purposes. This means that the earliest to latest arrival times are defined by the descent/diversion flight profile. The applicable time window should consider the earliest to latest expected arrival times for each en route alternate aerodrome based on the planned departure time. The validity period for a given alternate is typically determined based on a diversion from the first and last ETPs for the alternate.
And of course, weather at the alternate and on the flight there has to be planned for. “Weather planning factors help to define the suitable airfield from the adequate airfield,” Gribble said. “This is to allow for the potential of deteriorating weather conditions after the flight has commenced. Conditional forecast elements may also be defined — for example, a PROB 40 or TEMPO condition below the lowest applicable operating minima is normally taken into account.”
Once the operator diverts from the planned destination and heads to the alternate, the operator’s planning minimums dissolve, the alternate then becomes the new destination, and the published minimums of the alternate become the controlling factor. “There are planning factors based on how many runways and nav facilities are available; extended-range ops addresses this,” Gribble explained.
Inside the criteria for extended-range ops, “one piece of landing surface concrete with one navigation facility would require published minimums plus 400-ft. ceiling and published visibility plus 1 mi. to list this as a ‘suitable’ alternate,” he continued. “With no other guidance or insight, this would be a good place to start from as a reference point for Part 91 ops. Runway count points up another age-old question: With just one landing surface, is this one runway or two? Compare FAA OpsSpec C055 to ICAO Doc 9976 Flight Planning and Fuel Management (FPFM) manual.” When it comes to destination alternates, it’s easy to see that the basic idea from the standpoint of the FAA is that one landing surface is defined as two runways. But from the standpoint of ICAO, destination alternates will need two separate landing surfaces.
With two navigation facilities, the operator can reduce the weather planning fudge factor to published minimums plus 200-ft. ceiling and visibility plus 0.5 mi. “From this point, operating pilots need to loop back and consider the runway involved and tailwind/crosswind limitations,” Gribble said. “The lowest minimums published may not be for the runway you want to land on based on local wind conditions. This will drive you from a precision approach at 200 and a half to a non-precision approach at 600 and two.”
What about emergencies other than depressurizations? What happens if your airplane is on fire? “And that’s a whole other conversation,” Launius said. “Nothing else matters but getting on the ground. If you are on a long-range flight, some choices for a fire or an engine inop are more acceptable than those for a medical diversion in which you are looking for something more. The flight planners are not going to give you a tier of alternates; it’s up to you to go beyond what they give you for the situations that you might encounter. When you’re putting together your flight, you need to make your own tier of alternates, some for medical diverts and others for pure emergencies like engine loss, smoke and fumes, or an outright fire.”
There comes a time in long-range flight planning where, no matter the range capability of your aircraft, the austerity of your route will not support the necessity for accessible alternates in the event of a cabin depressurization — or other contingency. “The solution is often available,” Launius offered: “Plan an additional stop for fuel or a route that is not as direct. Using the polar example, you could take a more southerly route with lots of good alternates, but it will add 1:30 to the duration of the trip. We are pushing business jets with 6,500-nm range on routes with very limited options, like the polar routes. The airlines have longer-range aircraft and are not as concerned about it. An effective medical divert is what we’re talking about.”
Supplemental Oxygen: It Can Save Your Life
The FARs and ICAO generally align regarding supplemental oxygen, and once inside of ICAO Annex 6, there is a virtual reprint from the commercial side (Part 1) to the general aviation side (Part 2) which specifies that life-sustaining oxygen for a rapid descent is as critical as the fuel to get to a runway.
Part 91.211 discusses the same thing: supplemental oxygen for a general aviation operation. (The FAA published a change to Part 121.329 specifying that now, if one pilot leaves his or her station above FL 410, as opposed to FL 250 (the previous limit), the other has to put on a quick donning mask [which may seem counterintuitive].)
Just remember the cumbersome quick-don supplemental oxygen mask is your best friend if you are unfortunate enough to blow a cabin.
For Further Reading . . .
“Captain Eddie,” proprietor of the “Code 7700” website and BCA contributor James Albright’s nom de cyber, offers two commentaries on rapid cabin depressurization well worth the reading.
The first is a detailed primer on the structure of the atmosphere, flight physiology after loss of pressurization, tips on oxygen masks, timing the descent, and more.
The second is a fable on cockpit leadership and the wages of fooling around with pressurization controls when you don’t know what you’re doing.