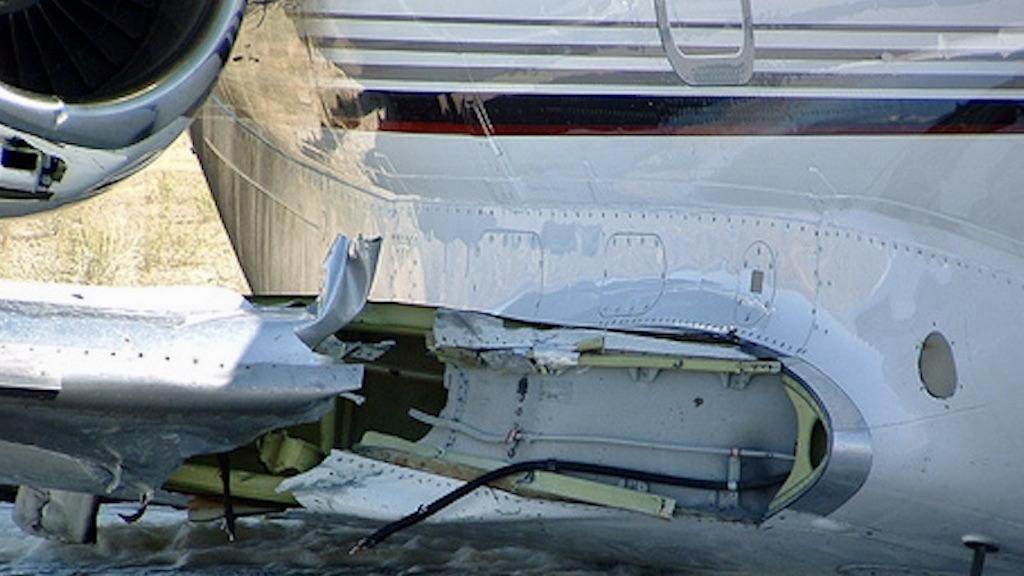
Unbeknown to the flight crew, the right wing of Hawker 800XP involved in a midair collision with a glider suffered immense damage, causing a large loss of lift and extra drag.
Expectancy means that we react more rapidly and accurately to situations in which we anticipate certain actions or events as opposed to a situation in which the events are a surprise. For example, the slightest abnormality in the aircraft’s performance or handling during a takeoff in the simulator is quickly interpreted as an engine failure. But the real world has many other different surprises.
This author’s lengthy search of NASA Aviation Safety Reporting System (ASRS) files found that only two of the 175 business jet high speed rejected takeoff (RTO) reports were caused by powerplant malfunctions. In fact, more than 96% of the high-speed RTO incidents in the NTSB, FAA and NASA ASRS records were due to non-engine causes. An important point from this data reveals that the real world has other surprises that are more likely to cause a high-speed RTO.
It simply isn’t feasible to train for all the possibilities that might cause a high-speed RTO. Additionally, the possible damage to an aircraft’s structure and handling characteristics following a high-speed tire failure (which is four times more likely to happen than an engine failure) or an animal strike (which is two times more likely) creates a bewildering number of factors that can’t be quickly turned into a simple Go/No-Go decision.
Abrupt Control Deterioration
A professional pilot needs to know an aircraft’s aerodynamic handling characteristics and limitations for all phases of flight, varying flap conditions, changes caused by environmental conditions, as well as the conditions that cause instruments to display misleading information.
A presentation entitled “Loss of Control,” given to the International Society of Air Safety Investigators in September 2011, revealed that 31 of 71 loss of control (LOC) accidents were due to unintentional stalls. Incidentally, aircraft were configured with gear and flaps extended (either partially or fully) in 52 of the 71 accidents. Furthermore, in the slower speed regime, the aircraft tends to be at a higher angle of attack that can further limit its maneuverability without stalling the aircraft.
Fully two-thirds (45 of 71) of the LOC accidents occurred within 1,000 ft. of the ground. This unfortunately means that most of the accidents occur with very little margin of safety. There are a troubling number of real-world factors that will cause a rapid stall without warning at an angle of attack (AOA) far below the critical AOA. Many of these phenomena will negatively change the aircraft’s behavior such that the aircraft is unrecoverable. In-flight conditions to include frost, ice, heavy rain, flap configuration, ground effect, cross winds, anti-icing fluids, deteriorated wing seals and the effects of Mach waves during transonic flight cause large reductions in a wing’s actual stall AOA.
High-performance airfoils exhibit stall behaviors completely different from low-performance airfoils, to include lack of aerodynamic warning, an abrupt loss of lift, and an uncontrolled rapid roll that cannot be arrested by flight control inputs. Deteriorated aerodynamic seals, especially those near the leading edge of the wing, cause a significant loss in the maximum lift of a wing as well as decrease the stall AOA. These airfoils are especially susceptible to any form of contamination. It is important to realize that the wing’s critical angle of attack may change with no apparent visual, tactile or performance cues associated with a contaminated condition.
The point is that training is giving the impression that stalls are relatively benign and can be easily recovered, when in fact real world conditions that are causing “stall by surprise” can occur rapidly, at AOAs far below the normal stall AOA, and that creates grave deterioration in the effectiveness of flight controls to respond. By the time the pilot is caught by surprise, the aircraft’s handling characteristics are already severely degraded, perhaps beyond the point of recovery. The safety implications and consequences of this misleading information are significant in upset prevention and recovery.
Primal Response
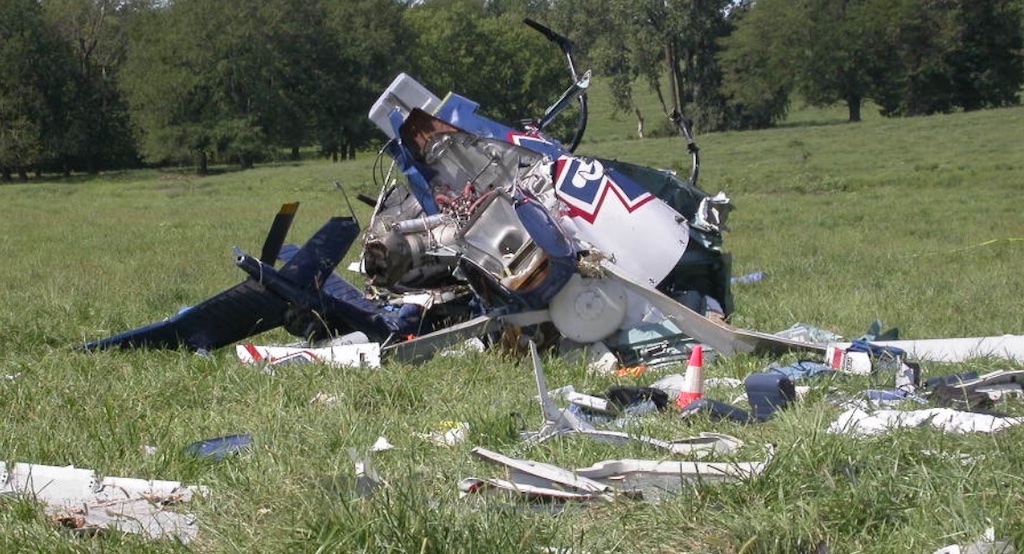
Some conditions require an immediate (and accurate) control input, or what is often termed primal response. The NTSB’s recreation of the Air Methods Eurocopter AS350 B2 helicopter accident near the Midwest National Air Center at Mosby, Missouri, on Aug. 26, 2011, illustrates this important point. The pilot, flight nurse, flight paramedic, and patient were killed.
Initially safety investigators were perplexed at how a highly trained former AH-64 Apache pilot failed to apply the proper autorotative flight control inputs after an engine failure due to fuel exhaustion.
During simulator recreations of the accident sequence, test pilots reacted to the flameout with simultaneous aft cyclic, down collective, and left pedal. The flameout was initiated at approximately 300 ft. AGL at a cruise airspeed of 115 kts. By utilizing these control inputs, the helicopter successfully landed, utilizing 27 sec. to transition into autorotation, bleeding off the kinetic energy during the cyclic flare, and setting down.
In contrast, when the simulator scenario attempted an initial pilot reaction with just the collective and no cyclic under the same entry conditions, time to impact was less than 5 sec!
The simulator flight tests concluded that when a loss of engine power occurs in the AS350 B2 at cruise airspeeds, the pilot must simultaneously apply aft cyclic and down collective to maintain rotor rpm. Additionally, these reactions are required within about 2 sec., or else the rotorcraft will be in an unrecoverable condition.
There are several important lessons from the Mosby accident. First of all, there are flight conditions in which the primal control inputs must be exactly correct for that specific make/model of aircraft, as the consequences of less-than-correct control inputs will further aggravate the loss of control.
Second, the correct control inputs must be made immediately, or else the condition of the aircraft will exceed the limits for recovery. Despite the credentials, experience and military training of the pilot, the training conducted in his transition into a civilian rotorcraft was ineffective.
This leads us to a third major point. Abrupt, unanticipated motions can overwhelm a flight crew. The sensory systems are poorly suited to abnormal accelerations and can overwhelm the body’s sensory, vestibular, proprioceptive (sense of body position and movement), and motor mechanisms. Some surprises, for example Vortex Ring State or loss of tail rotor control, will cause abrupt aircraft motions that can overwhelm the body and interfere with a pilot’s ability to focus vision and make accurate, fine-tuned appropriate corrective movements.
The fourth point highlights the pervasive nature of negative habit transfer. How do we train to instill the right “primal response” in a pilot when his or her background comes from aircraft with significantly different handling characteristics? Manufacturer Robinson Helicopter, found that highly experienced fixed-wing pilots habitually apply fixed-wing control inputs to disturbances that can produce mast-bumping contrary to the training they’ve received in helicopters.
Is it possible to “un-train” those primal responses learned over decades in one type of aircraft and implant a different set of primal responses? The Robinson data suggests perhaps not.
As we describe in Part 3 of this article, there can be severe consequences when aircraft don’t respond as expected.
Handling Surprises In The Real World, Part 1: https://aviationweek.com/business-aviation/safety-ops-regulation/handli…
Handling Surprises In The Real World, Part 2: https://aviationweek.com/business-aviation/safety-ops-regulation/handli…