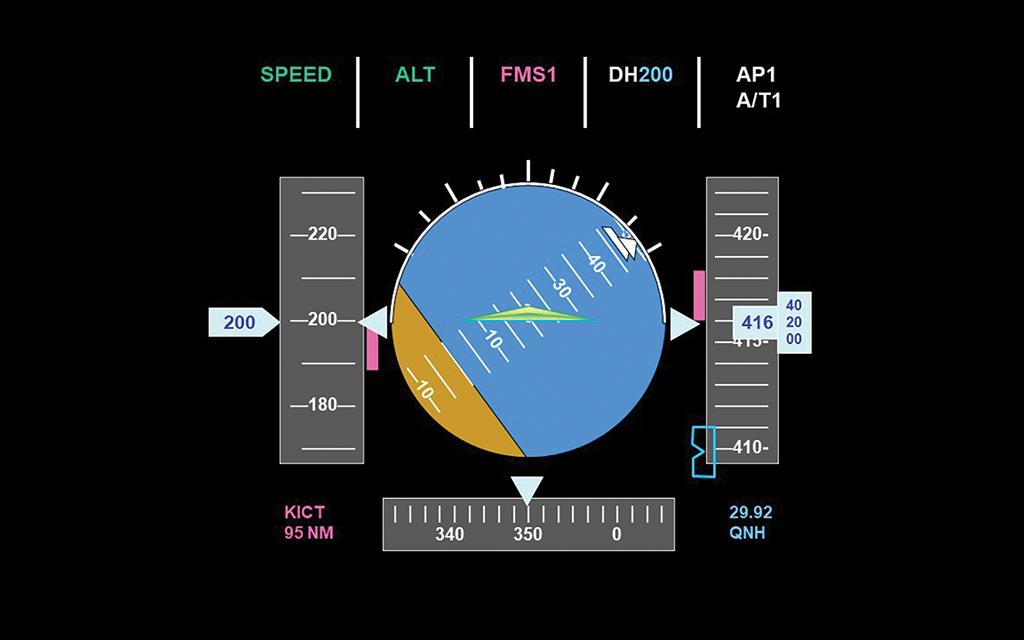
More than 20 years ago, Robert Agostino and David Ryan, then of Bombardier’s flight operations department, started an annual safety seminar in Wichita with the intent of teaching the company’s flight demonstration team pilots lessons learned from its flight test department. The Safety Standdown became an internal success and in 1999 Bombardier opened it to outside flight departments whereupon it grew into an industry-wide model.
While many subjects have been added to the Safety Standdown over the years, including fatigue management, cabin safety, water survival, flight physiology and high-altitude meteorology, the core focus of the program remains understanding the nuances of how test pilots probe the limits of aircraft performance and why understanding this process is essential to all pilots.
“The job of a test pilot is to eliminate risks,” says Agostino. “If specific risks cannot be eliminated, they must be identified, investigated and mitigated as much as possible.” That risk elimination/mitigation strategy, he says, is key to the safety of business flight operations.
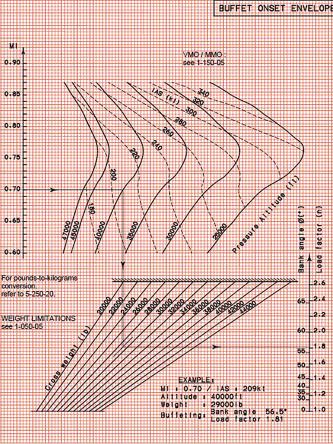
But identifying operational risks is difficult or impossible if pilots don’t know what they don’t know. Test pilots, for instance, probe the high-altitude flight envelope by performing a series of wind-up turns at various speeds, Mach numbers, altitudes and operating weights to determine a jet’s Mach buffet boundaries. This becomes relevant when operating at high cruising altitudes in turbulent air.
At high cruise altitudes, high-speed Mach buffet occurs when the local flow over the wing separates from the surface due to a Mach-induced shock wave. Low-speed Mach buffet occurs when the wing is operated at too high an angle of attack (AoA), accelerating the local flow over the wing to a Mach number that causes a shock wave strong enough to induce airflow separation.
The Mach buffet boundary diagram, also known as a buffet onset envelope chart, shows the indicated Mach numbers for various operating altitudes at which the wing has the highest spread between the low-speed and high-speed Mach buffet boundaries. The gist is that Mach buffet margins decrease at higher cruise altitudes and operating weights. But the optimum indicated Mach number for buffet resistance remains relatively constant regardless of altitude or aircraft weight.
Some flight departments print out the Mach buffet boundary diagram and include it as part of the dispatch release. They require their pilots use the chart together with forecast and real-time weather data during the mission to determine the highest altitude at which the aircraft can be operated safely in smooth air and also in varying degrees of turbulence.
Clear air turbulence gust loads easily can push a heavily loaded aircraft beyond its Mach buffet boundaries during high-altitude cruise, causing loss of stability, if not momentary loss of control. That’s an unnecessary risk that needs to be eliminated, says Agostino.
The buffet onset envelope chart shows pilots the maximum load factor the aircraft can experience without encountering Mach buffet at specific operating weights. In calm air or light turbulence, for example, the crew might choose to descend to an altitude where the aircraft has at least a 1.3g load factor available before Mach buffet at the optimum indicated Mach speed. In moderate to severe turbulence, it might be prudent to descend to an altitude where the aircraft has a 2.0g load factor margin to Mach buffet.
Agostino cites one west to east mission in the continental U.S. where the optimum initial cruise altitude for fuel efficiency was FL 470. However, there was an exceptionally strong subtropical jet stream flowing over the southern Rocky Mountains, potentially causing moderate to severe turbulence at high altitude downstream of the ridgelines.
That day, actual turbulence due to mountain wave east of the Rockies was considerably stronger than forecast. Thus, after crossing the Rockies and first encountering turbulence, Agostino elected to descend to FL 290 where the aircraft had a 2.0+g load factor margin to Mach buffet and where he found smooth air.
He credits using the aircraft’s Mach buffet boundary chart in making the decision to descend, rather than attempting to climb above the turbulence. At FL 490 to FL 510, he might have found smoother air, but the aircraft’s Mach buffet boundary load factor margins would have been razor thin.
Professional Training From Test Pilots
Flight Research Inc. (FRI) in Mojave, California, is one of few flight test organizations that offer formal classroom and flight training programs for business pilots that emphasize high-altitude aerodynamics, including stability and control characteristics, as well as high-altitude upset recognition and recovery training (URRT) in jet aircraft flying in the stratosphere. In contrast, several firms offer URRT in lightly wing-loaded, piston-engine airplanes at low altitudes where there is no opportunity to experience Mach effects or other high-altitude aerodynamic phenomena.
It’s the difference between finessing a 48,000-lb. intercity bus over an icy winter road and tossing around a 2,400-lb. sports car on a dry asphalt track at Laguna Seca. That’s one reason FRI teaches business pilots about Mach buffet boundaries, among other high-altitude flight regime topics.
Says Scott Glaser Ph.D., FRI’s senior vice president of operations, “Airplanes like to fly. That’s the ‘how’ and ‘why’ airplanes are designed. We, as pilots, may get into trouble because we may ask an airplane to do something it doesn’t want to do.” Professional jet pilots seldom intentionally exceed flight envelope limits. But it’s not difficult to exceed those limits inadvertently, if you’re not trained to recognize and mitigate the risk of high-altitude upset caused by weather or wake turbulence.
Glaser cites the near loss of a German-registered Bombardier Challenger 604 that encountered severe wake turbulence from an Emirates Airbus A380 that crossed 1,000 ft. overhead while the business jet was over the Indian Ocean in early January 2017. The wake turbulence from the 1.1-million-lb. mega jet caused the Challenger to roll more than 40 deg. to the right and then more than 30 deg. to the left, followed by vertical accelerations of 1.6g to -3.2g, according to flight data recorder information cited by the Flight Safety Foundation. Lateral acceleration reached 0.94g and pitch attitude plummeted from 9-deg. nose up to 20-deg. nose down. Airflow distortion caused the left engine to overtemp, prompting the crew to shut it down.
After plunging 8,700 ft. and rolling at least three times, the crew regained control of the aircraft at FL 250, restarted the failed engine and diverted to Muscat, Oman. One passenger suffered severe head injuries and a broken rib. Another passenger suffered a fractured vertebra. The other four passengers and flight attendant incurred minor injuries. The Challenger 604 was so severely damaged that it was written off as a total hull loss. Fortunately, all nine occupants aboard survived the encounter.
Glaser notes wingtip wake turbulence from jumbo jets can persist for several minutes. Elapsed time is no guarantee the wake turbulence has dissipated. The only sure defense is to recognize the threat and ensure your aircraft has generous altitude separation above the larger aircraft and/or ample lateral separation. The 1,000-ft. separation afforded in RVSM airspace provides no protection from wake turbulence when aircraft cross in opposite directions.
FRI officials note that the leading cause of fatalities in aviation is loss of control in flight, a risk that causes 25% more fatalities than controlled flight into terrain (CFIT) accidents and more than four times the deaths of midair collisions. They claim that loss of control accidents result in large part because few civilian pilots of high-performance aircraft receive URRT. As with the German Challenger 604 wake turbulence accident, a large part of URRT is identifying and steering clear of the upset risk, rather than simply reacting to the upset and making the appropriate power and control inputs to recover.
FRI’s four-day course includes comprehensive academics in ground school. But Glaser says it is simplified because the course is designed for professional business pilots, not test pilots. This contrasts with high-altitude aero ground school sessions at the first Bombardier Safety Standdowns that included esoterica such as Buckingham Pi theorem for determining lift and drag coefficients, the nuances of Reynolds numbers, determining pounds of drag due to Mach-induced shock waves and examining the oscillating superstall characteristics of a Saab SK-35 Draken fighter. However, business pilots in attendance told Bombardier they were not interested in enrolling in a test pilot school, so the hard science academics were toned down.
For the business pilot URRT program, Glaser shelved the complex test pilot school equations and non-relevant military aircraft aero review in favor of what’s most important in everyday business aircraft operations.
Five of the most relevant topics are AoA management, awareness of lift vector, aircraft stability characteristics above Mmo, high-altitude performance and stability degradation and maneuvering speed limitations, says Glaser.
Compressibility at high altitude, for instance, decreases stalling angle of attack (AOA) while increasing the indicated airspeed at stall. Indicated airspeed (IAS) at Mmo, though, decreases with altitude increase. The higher the aircraft flies, the lower the spread between IAS at stall and Mmo. Where the two slopes converge is known as the “coffin corner” in the flight envelope.
Virtually no current production business aircraft have such “coffin corners” in their flight envelopes. But the low- and high-speed limits of the diagram are calculated for smooth air operations. Real-world high-altitude turbulence can increase load factors, thereby momentarily squeezing together the low- and high-altitude IAS slopes. Gust-induced load factor effectively creates a “coffin corner” that’s not illustrated in the AFM flight envelope diagram. Effective AOA management only is possible by perusing several parts of the AFM, including the Mach buffet boundary chart and flight envelope diagram, along with the stalling speed chart that shows the effects of weight, load factor and high-lift devices.
AoA management is key to upset prevention. AOA reduction is crucial for stall recovery. It’s critical to be aware of stall hysteresis. Stall occurs as the wing reaches its maximum lift coefficient, an AoA where airflow is fully separated from the wing. But airflow will not reattach to the wing until AOA is reduced substantially below stalling AOA because of hysteresis. Some studies indicate that airflow will not reattach to the wing until AOA is reduced 40% below stall, depending upon the airfoil design. It’s critical to dump the nose, wait for the aircraft to accelerate and then nurse pitch attitude back to the horizon.
Moreover, most turbofan engines only produce 20-25% as much thrust at high altitude as they do at sea level, thus there’s no way to “power out” of a stall at altitude. The only effective way is to immediately reduce AOA, push up the throttles for what that’s worth and allow gravity to accelerate the aircraft to a safe stall recovery speed. Notably, it’s nearly impossible for line pilots to push precisely to a 0.5g load, even though that is recommended in some URRT syllabi. Instead, if pilots push until they’re light in their seats and dust starts to rise on the flight deck, they know they’re expediting stall recovery.
Yet, when in a nose-low stall recovery pitch attitude, it is critical to be aware of Mach buffet boundary limits. If the maximum load factor is exceeded when pulling up to a nose-level pitch attitude, the crew risks encountering a secondary stall.
Glaser says that is not the only aspect of high-altitude performance degradation of which pilots need to be aware. Aerodynamic pitch, roll and yaw damping are far less at high altitude than at sea level because air densities are 80-85% lower than at the surface. This makes it much more challenging to hand-fly the aircraft. In addition, RVSM altitude holding requirements all but mandate use of autopilot in cruise, denying pilots opportunities to practice their flying skills in thin air.
At high altitude, some aircraft, for example, exhibit impressively divergent lateral-directional oscillation (aka Dutch roll) characteristics when the autopilot is turned off and if the yaw damper fails. Others will exhibit divergent long-period pitch damping at high altitude, while they are well damped in pitch at low altitude. Those oscillations may be difficult, if not impossible to dampen with manual control inputs. Extending speed brakes and descending into denser air may be the only option.
The relationship between maximum operating speed and Mach number, maximum speed and Mach number for stability characteristics, and maximum demonstrated dive speed and Mach number are important to understand, especially in the context of high-altitude upset caused by turbulence gust loads, inadvertent control inputs, passengers moving about the cabin or other factors. The Mmo speed limit is set to provide positive pitch stability and adequate control margins up to Mfc, the maximum speed for stability characteristics. Speed increase up to Mfc must be accompanied by a linear, increasing push on the stick. In other words, speed increase above trim speed must be accompanied by a push to maintain the higher speed and a speed decrease must be accompanied by a pull to maintain the lower speed.
For most transport category aircraft, Mmo must be at least Mach 0.07 below Mdf, demonstrated dive Mach. Generally, aircraft are required to have positive pitch stability all the way to Mdf. But stick force can decrease to neutral between Mfc and Mdf. As Mfc can be as little as halfway between Mmo and Mdf, in some aircraft, stick force above Mfc can become uncomfortably soft with a speed increase as little as Mach 0.035 above Mmo. And if you exceed Mdf, there’s no assurance that shock-induced flow separation won’t result in a sudden aft shift in the center of pressure over the wing, causing the Mach tuck effect from which it may be impossible to recover.
Bottom line? Regard Mmo as a hard speed limit. If you inadvertently exceed it, make appropriate pitch, power and high-drag device inputs to slow the aircraft below Mmo to assure adequate stability and control margins.
Glaser’s program also includes a discussion of Va maneuvering speed. Some pilots believe that any control inputs they make below the Va limit will not cause structural damage. But as the American Airlines Flight 587 Airbus 300 accident during climb-out from JFK International Airport in 2001 proved, multiple full control inputs in one axis or simultaneous full control inputs in more than one axis can cause serious or fatal structural damage even though ultimate vertical load factor never exceeds 2.5g. Cyclic rudder inputs, for instance, easily can overstress a vertical fin, even up to the point of structural failure.
The FRI ground school covers other aspects of aircraft certification and how the flight envelope is defined, providing an understanding of limitations of test procedures required for airworthiness approval.
Flight Experience in Three Aircraft
FRI’s flight training program begins with test pilots first demonstrating, then client pilots practicing, URRT basics in a T-67 Slingsby Firefly aerobatic basic trainer. It’s useful for learning how to recognize and react to unusual attitudes and how to apply “push-power-roll-recover” attitude and speed correction techniques.
Clients then make the transition to the North American NA265 Sabreliner, an aircraft that has the high-altitude performance, wing loading, polar moments of inertia and control responses of a typical turbofan business aircraft. Flying the Sabreliner affords opportunities to experience high-altitude upset and practice recovering without exceeding Mach buffet boundary, Vmo or Mmo limits. The Sabreliner also enables clients to sample the reduced static and dynamic stability and spongier aerodynamic damping characteristics when hand-flying a business jet in the stratosphere.
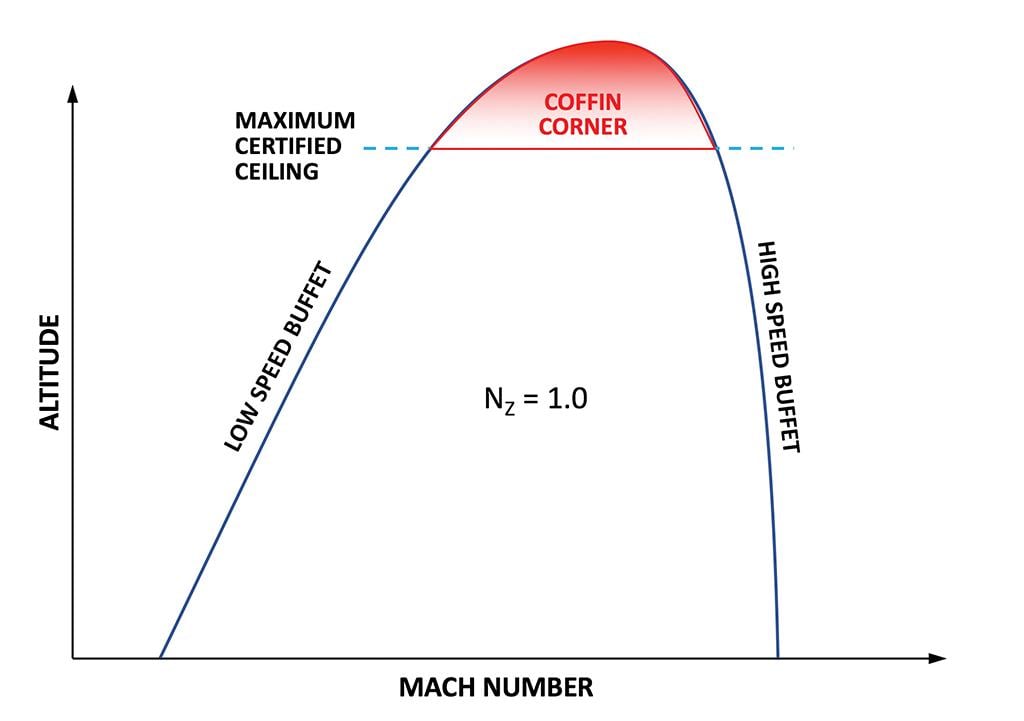
The final two flights are in the Aermacchi MB-326 Impala, a tandem-seat military trainer in which clients can experience full stalls, inverted flight, high G-load aerobatic maneuvers, and spin entries and recoveries. The flights in the Impala expose clients to high-performance maneuvers well in excess of what they would see in a business aircraft and are intended as confidence builders.
The take-away for professional business pilots is clear. Test pilots carefully, precisely and momentarily probe corners of the flight envelope to determine the aircraft’s structural and aerodynamic limits, thereby enabling designers to define safe operating speeds, Mach numbers and load factors that provide adequate margins if such inadvertent excursions are encountered. Inadvertently or intentionally exceed the aircraft’s limitation and you risk becoming an accident statistic. Fly within the limits and you’ll prolong the aircraft’s service life as well as provide the safest, most comfortable ride for all occupants.