Know Your Aircraft’s Specific Handling Characteristics, Part 3
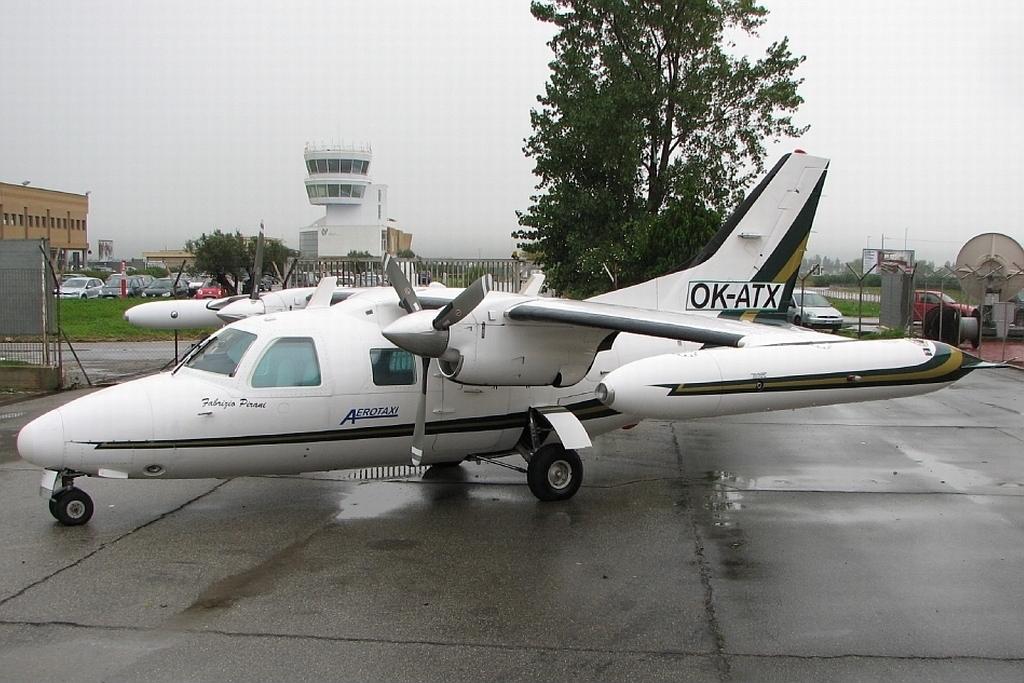
This is the third and final part of this article series on handling characteristics. The first part covers experience and the second part discusses differences between aircraft.
A prime example of the necessity of properly applying specific control inputs and procedures during a performance-demanding engine failure on takeoff is provided by the Mitsubishi MU-2. The aircraft’s design features fell under intense scrutiny after several high-profile accidents, including one that killed South Dakota Gov. George Mickelson in 1993. Advocates for the MU-2 voiced strong counter-opinions that the accidents were caused by pilots who were not adequately trained in its specific handling characteristics.
One of the unique design aspects of the MU-2 is the use of roll spoilers as opposed to conventional ailerons. Moving the yoke left will raise the spoiler on top of the left wing, which reduces lift on that wing, causing the aircraft to bank to the left. Sounds simple, right?
An engine failure on takeoff will instantly differentiate a pilot who is properly trained in the MU-2’s handling procedures from someone who isn’t. One of the clear differences will be the usage of the roll spoilers. Flight testing has demonstrated that a raised spoiler on the wing of the operating engine will reduce the single-engine climb.
The full-span, double-slotted Fowler flaps are highly effective on the MU-2, creating a 26-kt. margin in the stall between flaps up versus flaps down. Prematurely retracting flaps at low airspeed with an engine out can result in a complete loss of climb performance. MU-2 pilots are trained to leave the flaps alone after an engine failure until accelerating to at least the appropriate airspeed for the next flap setting.
Properly maintaining control, nailing the correct rudder input, and adjusting the servo tabs (the equivalent of aileron trim) to trim out the spoiler input can result in a fully loaded MU-2 safely climbing at 500 fpm on one engine.
In 2008, the FAA issued SFAR 108, which mandated type-specific training for pilots in the MU-2. In the 20 years before SFAR 108 was published, the MU-2-series aircraft had 80 accidents with 40 fatalities. Since the SFAR was implemented, the fleet of approximately 300 aircraft operating under FAR Parts 91 and 135 in the U.S. has experienced 15 accidents, of which five included fatal injuries. Under a newer rule, all training conducted in an MU-2B series airplane must follow an FAA-approved MU-2B training program that meets the standards set forth in a new Subpart N of Part 91.
Control Deflections Have Different Effects
The FAA’s Airplane Upset Recovery Training Aid reminds pilots that “Airplane upsets have occurred when the pilot has made incorrect adjustments…. If the pilot’s control inputs are reactionary, unplanned and excessive, the airplane reaction may be a complete surprise. A continued divergence from what is expected due to excessive control inputs can lead to upset…. Control deflections at one point in the flight envelope might not be appropriate in another part of the flight envelope….” A pilot may find that his own control inputs can cause unwanted motion that could lead to an upset or loss of control. Known as “pilot-induced-oscillation” (PIO), this condition occurs when a pilot’s commands become out of phase with the aircraft’s motion. When the aircraft doesn’t respond as quickly or as aggressively as the pilot desires, the pilot often responds with inputs that grow increasingly out of phase with the aircraft response.
On April 6, 1993, China Eastern Airlines Flight 583, a McDonnell Douglas MD-11, was cruising at FL330 near Shemya, Alaska, when its leading-edge wing slats inadvertently deployed, which caused the airplane to pitch up. The NTSB determined that the inadvertent deployment of the slats was the result of an inadequately designed flap/slat handle that allowed it to be easily and inadvertently dislodged from the up/ret position, thereby causing slat extension. The autopilot disconnected and the reactionary elevator control inputs by the flight crew in response to this initial pitch-up induced several violent nose-down and nose-up pitch oscillation cycles as it lost 5,000 ft. Unfortunately, the violent pitch oscillations caused two fatal injuries and 60 serious injuries due to the lack of seat restraint by the occupants.
The design of the MD-11 included “reduced longitudinal stability”--basically a relatively rearward CG to reduce trim drag during cruise flight, but in turn this means the aircraft has relatively light elevator control forces at high-altitude, high-speed cruise flight. Key to a successful recovery in this case is to recognize the extreme pitch sensitivity of the aircraft at high-altitude cruise flight.
In its causal statement, the NTSB found that contributing to the violence of the pitch oscillations was the lack of specific MD-11 pilot training in recovery from high-altitude upsets, and the influence of the stall warning system on the captain's control responses.
Differences in Flight Control Sensitivity
On Nov. 12, 2001, American Airlines Flight 587, an Airbus A300-605R, encountered wake turbulence two times from a Japan Air Lines Boeing 747 that had taken off from JFK International Airport before Flight 587. The effect of the turbulence was minor. However, the first officer reacted to this first wake turbulence encounter by moving the control wheel rapidly right and left several times, with large control wheel deflections up to 37 deg. right and 34 deg. left. The first officer made five subsequent alternating full rudder pedal inputs until the vertical stabilizer separated from the airplane. The A300's vertical stabilizer and rudder separated in flight and impacted in Jamaica Bay, about 1 mi. north of the main wreckage site. The airplane's engines subsequently separated in flight and were found several blocks north and east of the main wreckage site. All 260 people aboard the airplane and five people on the ground were killed. The airplane was destroyed by impact forces and a post-crash fire.
The NTSB’s airplane performance study revealed that the first officer’s alternating rudder pedal inputs led to increasing sideslip angles that produced extremely high aerodynamic loads on the vertical stabilizer. In fact, the four additional full rudder deflections (left-right-left-right) allowed the buildup of enough sideslip angle to produce aerodynamic loads on the vertical stabilizer that were about two times the loads defined by the limit load design envelope.
The NTSB investigation revealed a number of deficiencies in the American Airlines advanced maneuvers program (AMP). The rudder pedal sensitivity was significantly “boosted” in the 600 series of the Airbus 300. In the earlier series of the Airbus 300, the rudder pedal sensitivity at 250 kt. was 0.003 Gs per pound. In contrast, the rudder pedal sensitivity is seven times greater in the 600 series. The Airbus A300-600 rudder control system had the lightest pedal forces of all the transport-category aircraft evaluated by the NTSB. However, pilots weren’t adequately trained on the increased sensitivity of the rudder pedal inputs on the A300-600 at high airspeeds. The Safety Board’s investigation determined that “Pilots have generally had little exposure to, and therefore may not fully understand, the effect of large rudder-pedal inputs in normal flight or the mechanism by which rudder deflections induce roll on a transport-category airplane.” The Safety Board’s review of other carriers’ upset recovery programs indicated that the shortcomings in the AMP are not unique and that inconsistencies exist among programs.
These examples illustrate that each aircraft model has unique momentum characteristics, handling characteristics, flight control characteristics and limitations, system operating characteristics, structural limitations, and procedures for normal and abnormal conditions. A “standard” procedure or practice that applies in one aircraft can lead to catastrophic consequences in a different type of aircraft. It is vital to be informed and proficient in your aircraft’s specific handling characteristics.