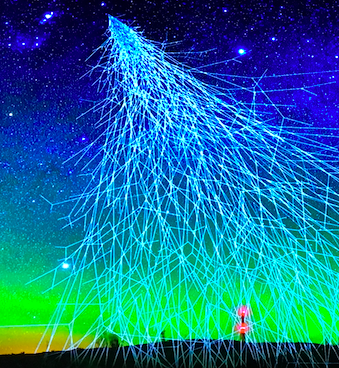
The first part of this article series examined how much is “too much” cosmic radiation exposure.
Concern over cosmic radiation exposure has surfaced in recent years, although due to its technical content the discussions have usually remained within a small group of specialists in aerospace medicine and radiation biology. At present, the biological effects of heightened exposure to radiation from neutrons are not fully understood. Furthermore, the exposure of a crewmember’s whole body during a transatlantic flight is diluted over several hours, rather than receiving the intense burst of radiation during an X-ray. There is considerable uncertainty within the respective scientific fields about the health risks due to the cumulative exposure to low-dose, high-energy cosmic radiation. This is partly a reflection of the immaturity of the scientific knowledge on this specific issue.
Our distinguished lecturers at CERN made a special point that workers in other industries who are exposed to far less radiation are required to follow strict rules for the mandatory usage of personal protective equipment, extensive shielding from the source of radiation, as well as monitoring.
In 1990, the International Committee on Radiological Protection (ICRP) recommended that jet air crews should be considered occupationally exposed to ionizing radiation. This has led to the introduction of corresponding exposure monitoring schemes in many jurisdictions. In Europe, air crews liable to receive more than 1 mSv per year must be assessed and informed of the potential risks with tactical adjustment of working duties expected as a response to exposures significantly greater than the average. The purpose of this is to ensure that no air crewmember will approach the internationally accepted limiting effective dose for all ionizing radiation of 20 mSv per year averaged over five consecutive years, or 50 mSv in any one year.
One particular area of agreement centers on the heightened risk to the fetus in pregnant females. The current accepted dose limit for pregnant crew members is 1 mSv during the declared term of the pregnancy. As a result of this, many airlines now have a policy of transferring pregnant air crewmembers to ground duties as soon as pregnancy is confirmed.
More Problematic in the Future
Several trends in aviation will make cosmic radiation a more-frequent risk factor. The growth in air traffic over the polar regions has been on a steep upward trajectory in recent decades. New city pairings that go over the polar regions are definitely at higher risk. Ultra-long-range aircraft are likely to spend considerable portions of their flight profiles at higher latitudes and longitudes. The cosmic radiation dose rate for a supersonic aircraft flying Great Circle routes at even, high altitudes will be problematic.
There are a number of practical difficulties when trying to apply conventional methods to limit a crewmember’s exposure to this radiation. Medical workers using X-ray machines take painstaking efforts to wear heavy protective shields, and to stand behind special protective structures. Adding protective structures to an aircraft isn’t feasible simply due to the weight. The physical burden of wearing protective clothing is unacceptable. Cruising at lower altitudes would result in much higher fuel costs, not to mention the undesirable aspect of creating a larger carbon footprint. Ironically, if the route of flight is longer, it would result in more radiation exposure. Less block hours spent in these higher-risk flight profiles is prudent, but from a staffing point of view, it will complicate crew scheduling.
The airlines, in collaboration with the manufacturers and regulatory agencies, have assembled extensive supplements to their operating specifications for polar flights. Cosmic radiation is just one of the many special considerations for polar flying. Space weather has a marked effect on aircraft in polar regions. This includes solar radiation storms, geomagnetic storms, ionospheric storms and solar flares. These can cause harm to humans, and negatively affect communications and navigation systems.
Once again, we are dealing with a highly scientific topic, and the terminology of the specialists in this field is not easy for aviation professionals to discern into practical information. This isn’t a flight region where you can sit through a two-day course and deem yourself to be qualified and knowledgeable. Planning for operations in polar regions must be comprehensive and it requires considerable expertise in a wide spectrum of polar issues to adequately prepare against the threats of this extreme environment.
One Final Note
The educational team leaders at CERN gave this Global Engagement group of 23 students a lifetime learning experience. The students left CERN’s campus with a deeper appreciation for the decades-long sustained and collaborative effort that is necessary in modern science. If you are involved with the education of tomorrow’s aviation workforce, a trip to CERN is a fabulous learning experience.