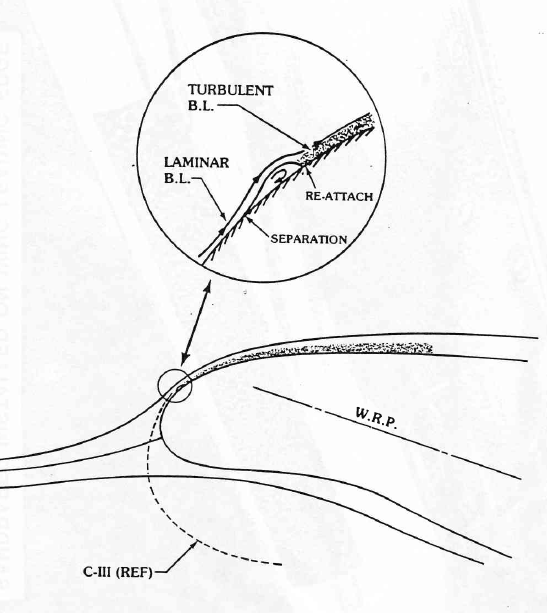
In Part 1 of this article, we discussed airfoil design and trailing-edge stall.
Even though you have been inundated throughout your flying career about the trailing-edge stall behavior of an airfoil, what is the likelihood that your high-performance aircraft utilizes an airfoil of this older design? Practically nil.
One inherent disadvantage of thicker airfoils is the propensity to develop a strong Mach wave due to the acceleration of air over the bulbous leading edge during transonic flight. This creates performance-robbing drag. Thus, with the advent of the jet era, aeronautical engineers began to utilize thin airfoils as a design method to lessen the development of Mach waves for aircraft that would cruise at transonic speeds.
The Learjet 23 was a product of this aeronautical concept. Its first flight on Oct. 7, 1963, signified the beginning of a revolution in transport aircraft to cruise at relatively fast transonic Mach numbers. It was designed with a 9% thick airfoil section, no wing twist, and the characteristic tip tanks.
While the potential cruise speeds of these jets were attractive, accidents and serious incidents revealed pilots’ lack of understanding of the different aerodynamics of airfoils designed for high-speed flight. As a result, they were ill-equipped to properly diagnose, prevent and recover from phenomena such as Mach tuck, deep stalls, aileron snatch and unforgiving abrupt stalls with uncontrollable wing roll-off.
In complete contrast to the traditional trailing-edge stall that is a characteristic of slower aircraft, flight tests by Lear observed that as the angle of attack increased, the flow around the tip tank caused a high AOA at the junction of the tip tank and the wing leading edge, which caused a triangular shaped area of separated airflow. This impacted the airflow over the aileron. The airflow over one wing would suddenly separate entirely, causing a rapid and large roll-off, often beyond 90 deg. of bank. Furthermore, there was little or no aerodynamic stall warning buffet. It was also noted that the stall characteristics deteriorated markedly with small accumulations of ice on the wing leading edges.
The rapid roll-off and abrupt stall with no aerodynamic warning caught pilots by surprise, adversely impacting recognition or recovery. An example of this occurred on Dec. 4, 1978, to a Learjet 25C that was on approach to Anchorage International Airport (PANC) with seven persons on board. Light to moderate icing was forecast in clouds below 12,000 ft. along with turbulence and gusting winds in the vicinity of the airport. The flight path was normal almost to touchdown, when the aircraft suddenly pitched up and began to bank steeply from side to side. The Learjet rolled to the right until its right wing struck the ground. Tragically, five of the seven occupants were fatally injured. One of the survivors of the violent impact was then Senate Minority Whip Ted Stevens, who had flown C-46s over the infamous “Hump” route in Asia during World War II and was a recipient of the Distinguished Flying Cross. Sadly, his wife of 26 years was one of the accident’s victims.
The NTSB determined the probable cause of the accident to be an encounter with strong, gusting crosswinds during the landing attempt, which caused the aircraft to roll abruptly and unexpectedly. The ensuing loss of control resulted from inappropriate pilot techniques during the attempt to regain control of the aircraft. Suspected light ice accumulations on the aerodynamic surfaces may have contributed to a stall and loss of control.
Flight Testing Uncovers the Leading-Edge Stall
In 1982, the Learjet flight-test team was tasked with trying to make improvements to the stall characteristics of its earlier aircraft. One of its first challenges was to better understand these abrupt stall characteristics. Earlier NASA studies had discovered that airfoils with this design exhibited a smooth laminar flow over the leading edge at low AOAs. As the AOA is increased, an adverse pressure gradient increases to the point where a small amount of separation occurs near the leading edge. This separation causes the boundary layer to transition from laminar to turbulent, and at this point the airflow would form a small rotating “bubble” that helped to reattach the boundary layer to the airfoil. This bubble remains attached to the airfoil until the AOA is increased to a critical value, whereupon it suddenly breaks down for reasons not presently understood. In smoke wind-tunnel tests the bubble appears to burst. At this point the boundary layer fails to reattach to the airfoil, causing very rapid full chord airflow separation. In other words, this causes a completely separated boundary layer, i.e., a stall, over the entire airfoil. Furthermore, traditional symptoms of stalls such as unresponsive flight controls or aerodynamic buffeting are not present.
Flight-test teams led by Learjet’s legendary test pilot Pete Reynolds utilized small tufts of yarn across a wing to observe its boundary layer behavior. These teams noticed that the burst of this leading-edge bubble along a small portion of the wingspan will instantly extend full span and the entire wing can separate almost instantly.
The leading-edge stall is common on airfoils with medium thickness, between 10-16%, utilized on high-performance aircraft. How can you tell if your aircraft uses an airfoil that exhibits the leading-edge stall? First look up the airfoil section for your wing with a quick internet search, and then search for the “lift curve” for that airfoil. An airfoil that exhibits classic leading-edge stall behavior, such as the NACA 4412, has little or no rounding of the lift curve near maximum lift. The lift coefficient exhibits an abrupt loss of lift. This differs from the other types of airfoils where the loss of lift is gradual after stall.
By the way, this information hasn’t been hidden from the aviation profession in secure vaults. Reynolds disseminated it throughout the professional journals so that the rest of the industry could gain useful insight. One example is his Ten Years of Stall Testing, published through the American Institute of Aeronautics and Astronautics as AIAA Paper #90-1268.
In the final part of this article, we’ll discuss the effects of wing leading-edge contamination on the stall AOA.