GE Details Sixth-Generation Adaptive Fighter Engine Plan
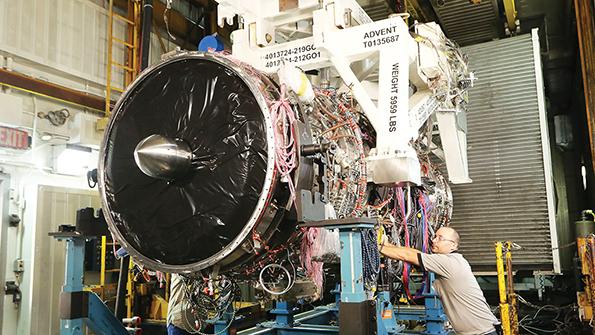
Credit: GE
U.S. military planners have now broadly accepted that the only way to meet the advanced performance needs of “sixth-generation” combat aircraft, barring changes to the laws of physics, will be the adoption of variable-cycle, or adaptive engine, technology. The future fighter will be required to not...
Subscription Required
This content requires a subscription to one of the Aviation Week Intelligence Network (AWIN) bundles.
Schedule a demo today to find out how you can access this content and similar content related to your area of the global aviation industry.
Already an AWIN subscriber? Login
Did you know? Aviation Week has won top honors multiple times in the Jesse H. Neal National Business Journalism Awards, the business-to-business media equivalent of the Pulitzer Prizes.