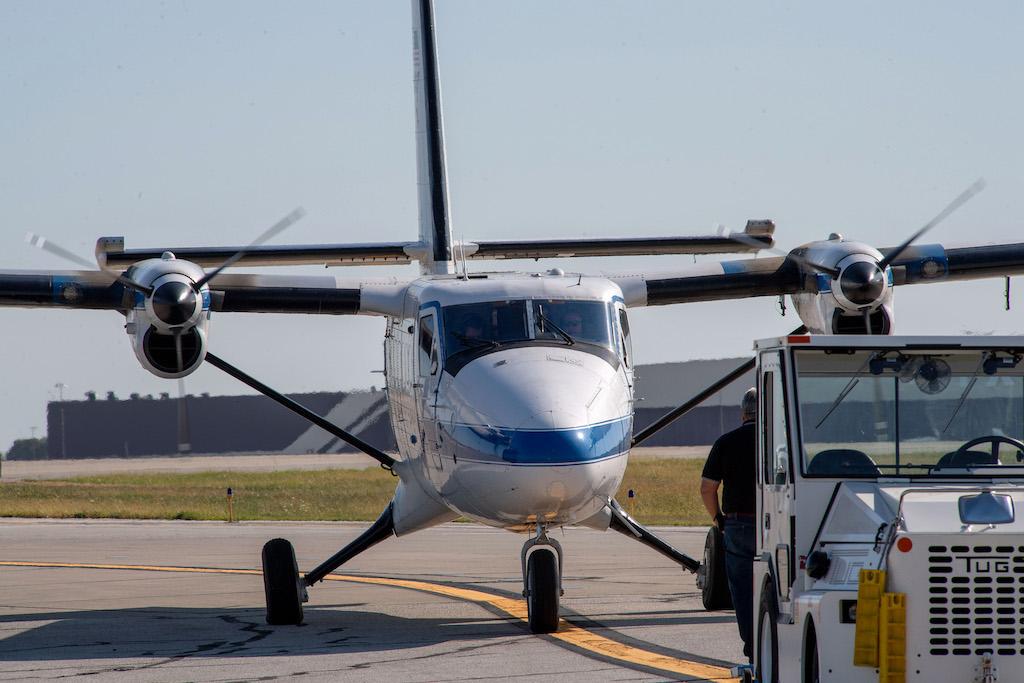
NASA’s former DHC-6 Twin Otter research aircraft.
I wrote on the topic of ice-contaminated tailplane stall a while back and apparently did not understand the problem. It is now years later and I still feel the same as I did then. I listen to, read about or watch videos concerning icing in aircraft and follow along just fine—until the part about “in an ice-contaminated tailplane stall, the recovery is just the opposite.” At that point, I always feel like a bike rider driving into a field at highway speeds.
My flying these days is done in a flying club. I can avoid icing by just not flying in clouds where the temperature may be conducive. The great thing about aviation in retirement is “heck, let’s just wait till the weather improves” is a scheduling option.
But in that club we instruct many new, low-time pilots. They range in background from high school students to highly successful businesspeople. A common characteristic of all the students is the willingness to absorb any information given by a higher-time pilot. That fact is a discussion point of constant consideration by club instructors. Negative training can occur whenever ideas or solutions are offered that can be misunderstood.
When BCA’s Roger Cox first wrote about wing and/or tail plane icing, I took a keen interest. Cox wrote about negative stall training in simulator lessons, something those of us who flew jets in the last 50 years have all experienced. Stalls were induced to first indication, then powered out of while maintaining altitude. The goal was stall recognition and recovery, but the emphasis became smooth and level flight throughout the maneuver. It looked good in the sim, but could have led to disaster in the real world.
Negative training has become a sort of buzzword at the flying club. Keep instruction simple, straightforward and easy to implement, and most importantly, help accomplish the desired outcome.
A Quick Review
If we assume a constant altitude (air density) and a constant size of the wing (planform area), there are only two variables involved in the creation of lift. These are the angle of attack (AOA) of the airfoil and the airspeed. A higher AOA means there is a higher coefficient of lift and more lift being produced at a given airspeed.
Eventually, as AOA continues to increase and flow begins to separate from the rear of the wing, the airfoil reaches its critical AOA. Beyond that, lift is being inversely produced. That is, the higher the AOA beyond the critical angle, the less lift the airfoil is producing. Eventually, no lift may be produced by the wing.
We all know that critical or stall AOA is the only constant when discussing uncontaminated airfoil stalls. The so-called “level flight stall speed” of an airplane is only the stall speed in level flight at a constant altitude, with no bank angle and at given weight. Change any of those variables, and the airfoil will stall (reach its critical AOA) at a different indicated airspeed.
For instance, at 60 deg. of bank, the wing has to produce twice as much lift as at level flight, just to keep the airplane level. This is because in a turn the lift vector is tilted and not all the lift is working against gravity. The wing will stall at the same angle, but at a higher airspeed because more total lift is required. Anything that increases AOA on an airfoil brings it closer to the airfoil stalling.
Center-Of-Gravity Effects
In flight, with center of gravity forward of the center of lift, the wing lift tends to push the nose down. This fact is counterbalanced for level flight by having the tail surface “lift down.” In other words, the tail is an airfoil that is on the airplane upside-down. At a constant airspeed, anything that calls for more lift down force is increasing the AOA on that tail plane.
As flaps are extended, increasing the wing lift and moving the center of lift even further aft, this has to be counteracted by having the tail surface produce more down force (lift). As we stated above, if air speed stays constant, the only way to increase lift is by increasing AOA. By asking the tail to produce more down force, we are increasing the AOA on the upside-down airfoil that is the horizontal stabilizer.
The downflow from the flaps, in airplanes susceptible to tail plane icing, is also creating more AOA on the tailplane. Within center-of-gravity and airspeed limits, and on uncontaminated airfoils, this is all designed into the airplane.
An Upside-Down Wing
A NASA video on DHC-6 Twin Otter tail plane icing states that when a wing stalls, the way to recover is to decrease the wing AOA. The same is true of any airfoil, even the upside-down airfoil of the tail plane. If it stalls, the AOA has to be reduced.
While flow separates from the rear to the front under normal circumstances, the video shows that icing on the tail plane leading edge also caused flow separation at the front of the tail airfoil. The video says this creates a negative pressure (separation flow) that is moving aft, thus the tendency of an ice-contaminated tail stall to seem to snatch the yoke forward. But the tail is also stalling “in the normal manner” with separation from the rear of the surface. The NASA video demonstrates this.
The U.S. FAA has issued guidance on which airplanes should be considered liable to be affected by negative tail plane stall characteristics. The NASA video goes to great lengths to explain the guidance is aimed at Twin Otter-type airplanes with high engines, big flaps and manual controls. Nevertheless, it also states, “many large and small general aviation aircraft are also susceptible to ice-induced tail plane stall.”
That statement seems to put just about everyone into the fray when it comes to tailplane icing—thus the need for instruction.
It is time for a simple “Approach In Icing Conditions” procedure, we argue in Part 2 of this article.