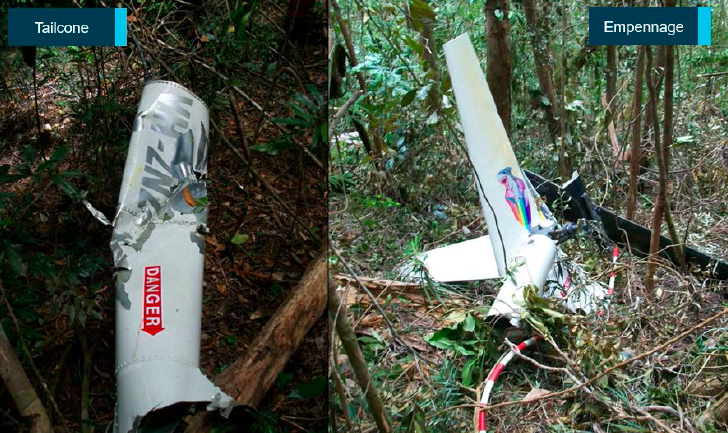
There are many sectors of rotorcraft aviation that regularly operate in the mountain environment. For the emergency medical services sector, it means dispatches into foreboding terrain, often when threatening weather is likely. For pilots involved with natural resource management, it might mean being dispatched with heavy loads of retardant to drop on a rapidly developing wildfire.
Others with specialized skills in external loads are employed installing construction components for power lines, ski lifts or other infrastructure on steep cliffs that would otherwise be inaccessible. Rotary-wing aircraft are used extensively by aerial tour companies in mountainous regions. And, of course, well-heeled powder skiing addicts will pay hefty daily prices for a helicopter to drop them on a knife-edge ridgeline so they can ski untracked powder chutes in terrain that otherwise would be inaccessible.
Flying in this environment is filled with meteorological risks that can be unforgiving. High-density altitudes, abrupt changes in winds, localized weather and turbulence all combine synergistically to shave safety margins razor-thin. Mast bumping, main-rotor stall, compromises in control and/or performance of the rotorcraft, and external load entanglement are serious undesired aircraft states caused by turbulence that must be avoided.
Australian media personality Graeme (“Shirley”) Strachan was a helicopter enthusiast and decided to do a training navigation flight in an Agusta 47-G helicopter in the mountainous terrain of Queensland on Aug. 29, 2001. When he didn’t return to the flight school, a search was initiated, and they eventually found the wreckage on the slopes of Mount Archer.
“The extensive damage to the helicopter, severed tailboom and the location of parts on the ground led transport safety investigators to conclude that the main-rotor blade may have contacted the tailboom in flight,” Air Safety Deputy Director Alan Stray said.
According to the Australian Transport Safety Bureau’s (ATSB) report, “This type of damage was consistent with flying into mountain wave turbulence, and may have occurred from one of two events: blade flapping [divergence of the main-rotor blade from its normal plane of rotation encountered during severe turbulence] or the pilot’s instinctive reaction to pull up after a sudden nose-down pitch from a change in the helicopter control input.… Weather conditions at the time were conducive to mountain waves on the northeast slope of Mount Archer near where the wreckage was found.”
According to Flight Safety Australia, a publication of the ATSB, “Encounters with mountain waves have led to catastrophic events in the past and pilots needed to be highly aware of their potentially deadly effects when interpreting weather forecasts and planning flight over mountainous terrain.… It is absolutely essential that aviators are aware of the wind and its potential effects on aircraft.”
“We hope that out of this tragedy, a greater pilot awareness of mountain waves will save lives in the future,” Stray said.
According to the FAA’s Basic Helicopter Handbook (AC 61-13B), Turbulence will be found in the following areas when wind velocity exceeds 10 mph:
(1) Near the ground on the downwind side of trees, buildings, hills or other obstructions. The turbulent area is always relative in size to that of the obstacle, and relative in intensity to the velocity of the wind.
(2) Near the surface on the immediate upwind side of any solid barrier such as trees in leaf, and buildings. This condition is not generally dangerous unless the wind velocity is approximately 20 mph or higher.
(3) In the air, above and slightly downhill of any sizable obstruction, such as a hill or mountain range. The size of the obstruction and the wind velocity govern the severity and the height to which the turbulence extends.
According to the U.S. Army Field Manual 3-04.202, Environmental Flight, the winds associated with mountains can be broken down into three main categories: prevailing winds, local winds and surface winds. The prevailing winds are those you would find on a national weather forecast or in the “winds aloft” forecast. It is these winds that are responsible for creating mountain waves.
Mast Bumping
Mast bumping is a condition that may occur with a teetering rotor system when excessive main-rotor flapping results from low “G” (load factor below 1.0) or abrupt control input. A low “G” flight condition can result from an abrupt cyclic pushover in forward flight. High forward airspeed, turbulence and excessive sideslip can accentuate the adverse effects of these control movements. The excessive flapping results in the main-rotor hub assembly striking the main-rotor mast with subsequent main-rotor system separation from the helicopter.
According to the FAA’s Rotorcraft Flying Handbook, “Since a low G condition could have disastrous results, the best way to prevent it from happening is to avoid the conditions where it might occur. This means avoiding turbulence as much as possible. If you do encounter turbulence, slow your forward airspeed and make small control inputs. If turbulence becomes excessive, consider making a precautionary landing. To help prevent turbulence-induced inputs, make sure your cyclic arm is properly supported. One way to accomplish this is to brace your arm against your leg.”
A 1998 accident involving a Robinson R22 further illustrates this threat. On Feb. 26, 1998, the R22 departed a private airport near Adelanto, California, on an FAR Part 91 personal flight. Witnesses reported that winds at the time of the accident were westerly at 20-25 kt. One witness reported he heard a loud “schwapp-like” noise followed shortly by engine silence, and then saw the helicopter disappear from view in a near-vertical descent. The helicopter impacted the ground near Littlerock, California, fatally injuring the sole occupant.
A replay of recorded radar data at High Desert TRACON showed the helicopter flying from east to west at 3,800 ft. when it disappeared from radar at the accident location. Approximately 5 min. earlier, the radar displayed the R22 about 7 mi. east of the accident location at 3,800 ft. MSL, at 80-kt. ground speed. A few minutes later, its ground speed had increased to 90 kt., then shortly thereafter to 130 kt. In the two subsequent radar returns, the ground speeds displayed were 140 and 120 kt. Ten seconds later, the helicopter had climbed 100 ft. and slowed to 80 kt. Radar returns of the aircraft were lost at this point.
A meteorology factual report prepared by the NTSB staff meteorologist reported that surface observations near the time of the accident from surrounding stations reported westerly to northwesterly surface winds at 10 to 30 kt. Winds aloft from the accident elevation to 10,000 ft. were northwesterly from 6 kt., increasing to 40 kt., and several pilots reported moderate to severe turbulence below 8,000 ft. with winds aloft of up to 50 kt. and updrafts and downdrafts near the mountains. AIRMET TANGO 3 was valid at the time of the accident for occasional moderate turbulence below 12,000 ft. MSL.
The post-accident investigation revealed that one main-rotor blade had entered the cockpit through the left windshield half and cut the cabin floor aft to the left seat. The location of an indentation in the cyclic-control cross tube, made by the rotor blade, corresponded to the cyclic control having been about in the full-left control position.
The NTSB determined the cause of the accident was the pilot’s improper use of the rotorcraft cyclic control in response to encountering terrain-induced turbulence. A factor in the accident was the pilot’s failure to obtain a preflight weather briefing, which contained precautionary notices for turbulence.
Not long before this accident, on April 2, 1996, the NTSB adopted a Special Investigation Report following the investigation into R22 and R44 accidents involving loss of main-rotor control and divergence of the main-rotor disk. The report included a finding that the cause of the loss of main-rotor control in many of the accidents “most likely stems from a large, abrupt pilot control input to a helicopter that is highly responsive to cyclic control inputs.”
In January 1996, Airworthiness Directive 95-26-04 was issued to owners of R22 helicopters requiring additions to the aircraft flight manual (AFM). The Emergency Procedures Section contains the following warnings:
Flying in high winds or turbulence should be avoided, but if unexpected turbulence is encountered, the following procedures are recommended:
(1) Reduce airspeed to between 60 or 70 KIAS.
(2) Tighten seat belt and firmly rest forearm on right leg to prevent unintentional control inputs.
(3) Do not overcontrol. Avoid large or abrupt control movements. Allow aircraft to go with the turbulence, then restore level flight with smooth, gentle control inputs.
(4) Leave governor on and do not chase rpm or airspeed. Momentary rpm or airspeed excursions are to be expected.
(5) Avoid flying on the downwind side of hills, ridges or tall buildings where the turbulence will likely be most severe.
(6) Never fly into a blind or box canyon during high winds.
These general recommendations mimic those contained in the FAA’s Rotorcraft Flying Handbook and Basic Helicopter Handbook.
Lest you mistakenly assume this is a problem restricted to just R22 helicopters, other helicopter types have suffered similar fatal main-rotor mast fracturing. Returning to base after a round-trip passenger flight on May 28, 1987, near Shelton, Washington, having disembarked passengers, a Hiller UH-12E was observed proceeding at high forward speed in strong but intermittent wind conditions. The rotorcraft impacted terrain, fatally injuring the pilot.
The investigation revealed that the main rotor separated in flight due to mast bumping, a phenomenon associated with teetering rotor systems, induced by exceeding the normal flight envelope for the aircraft. The NTSB determined the probable cause was total failure of the main-rotor mast and separation of the rotor system. Contributing factors included windshear and turbulence.
Main-Rotor Stall
On July 2, 1993, witnesses in San Francisco observed a Bell 47G2-M helicopter pitch up and then nose-over to impact. The sequence was characteristic of a retreating blade stall. The prevailing wind was reported to be extremely variable at about 50 mph. The accident report noted that from 15:30 to 16:30, 25 airplanes, mostly large airline transport category aircraft, had executed missed approaches at San Francisco International Airport due to the severe wind-shear conditions. One occupant of the Bell 47G2-M was fatally injured and the other was seriously injured. The NTSB determined the probable cause was the pilot’s inadequate preflight planning/preparation, which resulted in flight into high winds, turbulence and windshear.
An R44 was on a charter flight with one pilot and one passenger over Mount Windsor National Park near Mossman, Queensland, Australia, on Nov. 16, 2016, when one of the main-rotor blades struck and separated a section of the tailcone, which resulted in a near-vertical descent through the forest canopy.
The wreckage indicated that there was low engine power and rotor speed at the time of the strike, which was likely the result of a main-rotor-blade stall event. The ATSB also found that the R44 was likely operating in at least moderate turbulent flight conditions. The turbulence was associated with a 15- to 25-kt. wind flowing over the undulating terrain at a high density altitude.
Main-rotor stall is another adverse condition that becomes exacerbated in turbulence. Any flight condition that creates excessive angle of attack on the main-rotor blades can produce a stall.
According to the FAA’s Basic Helicopter Handbook, “The severe vertical gusts produced by turbulence can cause a sudden increase in angle of attack, resulting in increased rotor blade loads that are resisted by the inertia of the helicopter.”
Low main-rotor rpm, aggressive maneuvering, high collective angle (often the result of high-density altitude, over-pitching [exceeding power available] during climb, or high forward airspeed) can cause main-rotor stall, ultimately resulting in contact between the main rotor and airframe.
***End of Part 1***
See Helicopters In Turbulence Part 2 in the Sept. 8, 2021 edition of BCA Digest.