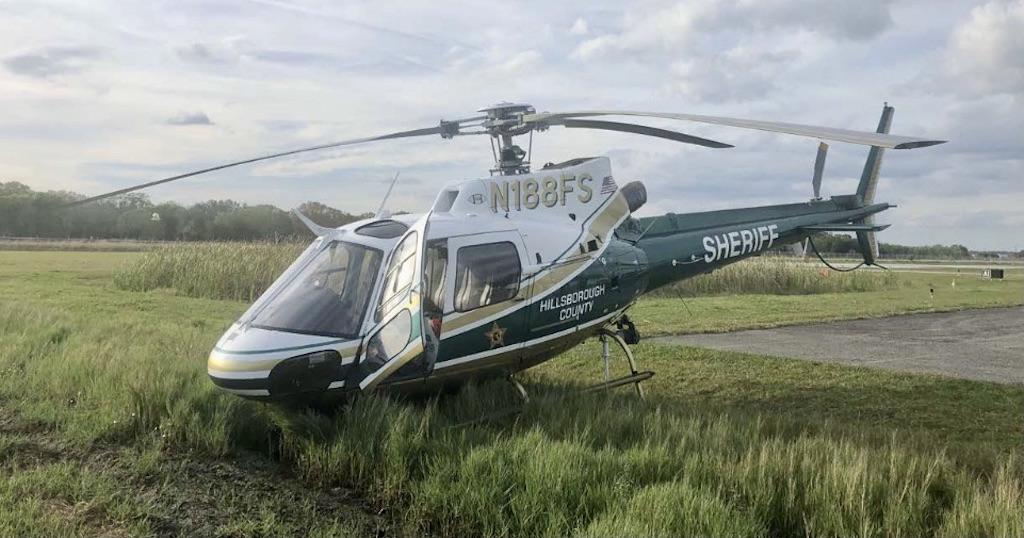
An instructor inadvertently moved the throttle lever from idle aft toward the 'off' position, which resulted in a total loss of engine power. The instructor took the controls, conducted a power-off autorotation, and landed the helicopter on the taxiway. It skidded about 180 ft, departed the taxiway onto adjacent grass, and came to rest in a drainage ditch.
How do we provide sufficient training to pilots to make certain they are proficient in the complex emergency procedures in a rotorcraft under a variety of real-world conditions?
Even though modern, properly maintained powerplants are reliable, a helicopter pilot must always be prepared to properly execute an autorotation. The U.S. Joint Helicopter Safety Analysis Team (JHSAT) “Compendium Report” (2000, 2001 and 2006) determined that autorotations, both actual emergencies and during training, occurred in 32% of the 523 accidents during that three-year period.
An autorotation is a complex perceptual motor skill. Unrecoverable consequences can quickly occur if the pilot’s inputs are incorrect, insufficient, excessive, or poorly timed. The only way to remain proficient is to practice this critical maneuver frequently. Ironically, the safety margins are so thin that sometimes the practice maneuver terminates in an accident. The JHSAT’s report noted that 68 of the 523 accidents, or 13%, occurred during autorotation training.
There is no debate within the industry on the importance and necessity of autorotation training. Industry and government working groups have issued numerous advisories to correct some of the common deficiencies. These include recommendations on safety briefings, throttle usage, prudent selection of practice areas, and the necessity to rapidly place the rotor tip-path-plane into a proper orientation for the autorotation.
Have these recommendations been effective? Perhaps. The following is a review of autorotation accident data from a recent four-year period to provide a comparison against trends from 15 years ago.
A total of 73 helicopter accident investigation final reports involving an autorotation were reviewed in the NTSB’s accident database; they occurred from the 2019 through 2022. Seven accidents resulted in 11 fatal injuries. All other accidents involved non-fatal injuries. Thirty of the 73 occurred during autorotation training.
An actual loss of power occurred in eight of the 30 autorotation training accidents. Thirty-eight of the 73 accidents involved turbine-powered helicopters. The rest occurred in piston-powered helicopters. Now let’s dig deeper into the data for important trends.
Insufficient Speed-Altitude Combination

Many important helicopter missions are conducted relatively close to the ground and at slow speeds. This is a necessity for the aerial firefighting pilot trying to drop a bucket of water precisely on a smoldering snag, as well as for the pilot hovering mere inches from high-voltage cables as a technician conducts electrical line maintenance. Unfortunately, 22 of the accidents reviewed for this report occurred at an insufficient altitude to adequately establish a steady state autorotation to avoid impact with terrain.
This happened to the pilot of a Bell UH-1B who was conducting helilogging operations on March 8, 2019, near Forks, Washington. Shortly after his first external-load lift of the day, company employees heard the pilot call mayday. The helicopter was found inverted in the designated logging area with the cargo line still attached along with a log. The pilot was fatally injured.
According to the NTSB accident report, the helicopter operator had not kept accurate documentation of engine cycles. The helicopter’s electronic cycle counter revealed that the sealing disk had exceeded its published life limit of 6,900 cycles. The cycle counter had recorded 12,023 cycles.
The NTSB determined the probable cause to be the total loss of engine power due to the failure of the gas generator sealing disk, a result of the operator’s exceedance of the sealing disk’s life limits at too low an altitude for the pilot to accomplish a successful autorotation that resulted in the collision with terrain.
There are combinations of initial altitudes and airspeeds from which a safe autorotational landing will be extremely difficult to perform if an engine fails during this precarious phase of flight. The boundaries of these combinations define the height-velocity diagram. The H-V diagram depicts two areas to be avoided: the low-airspeed/high-hover altitude (low flight altitude) region and the high-airspeed/low-altitude region. From these combinations it is almost impossible to demonstrate safe autorotative landings at a vertical touchdown speed within the design limits of the landing gear.
Many helicopter missions require extensive periods of time spent precariously close to the margins of the H-V diagram, so from a practical standpoint it isn’t realistic to tell pilots to always avoid flight within the shaded areas on the H-V diagram.
In addition, some helicopter missions are conducted in an airborne environment that is hazardous to turbine engine operations. Five prominent parameters in the wildfire airborne environment place abnormal stresses on turbine engines. First, while the “average” air temperature around wildfire incidents tends to be excessively hot, localized plumes of super-heated air rise from the flames. Additionally, radiant heat from the flames elevates air temperatures near the flame front, far above the “ambient” temperatures.
Secondly, the air from burning wildland fuel is a complex mixture of gases and aerosols. Thirdly, wildfires also emit large amounts of fine and course particulate matter (PM). Fourth, the air adjacent to the flame front is turbulent. Turbulent air flow through the engine inlet increases the chances of compressor stall and/or engine surges. Fifth, common flight maneuvers necessary for firefighting near the flame front increase the chances of hot gas ingestion into the engine inlet. All five of these conditions exist simultaneously and directly compromise the safety margins of turbine engines.
The historical record illustrates razor-thin safety margins in this environment. A Flight Safety Foundation study of helicopter accidents involved in aerial firefighting examined U.S. accident reports from 1974-98 involving helicopters engaged in aerial firefighting.
Thirty-four of the 97 accidents were caused by powerplant malfunctions and failures. Thirty-one of the 34 accidents resulted in severe injuries or worse to the occupants due in combination to an engine malfunction near the margins of the H-V envelope as well as the inhospitable terrain underneath the aircraft.
Observing operating limits, following a manufacturer’s maintenance schedule, utilizing the manufacturer’s guidelines for normal and all-weather operations, and engine trend monitoring are important elements to maximizing engine reliability, all of which are vital when the consequences of an engine failure don’t allow a safe autorotative landing.
Flare
The substantial damage in nine autorotation accidents reviewed by the author was attributed to hard landings. Improper flare technique was specifically cited in seven accidents, five of which occurred during power-on recoveries. Four accidents involved loss of rotor RPM, and all of these occurred in the final moments of a landing flare.
Every autorotational flare will be different depending on the existing wind conditions, airspeed, density altitude, the specific make and model of helicopter, and the rotorcraft gross weight.
The “FAA Helicopter Flying Handbook” (FAA-H-8083-21A) states in part: “Care must be taken in the execution of the flare so that the cyclic control is neither moved rearward so abruptly that it causes the helicopter to climb nor moved so slowly that it does not arrest the descent, which may allow the helicopter to settle so rapidly that the tail rotor strikes the ground. [E]xtreme caution should be used to avoid an excessive nose-high and tail-low attitude below 10 ft. The helicopter must be close to the landing attitude to keep the tail rotor from contacting the surface.”
Other errors included misjudging a proper height above the surface to begin the flare. This latter point is dependent on the pilot’s accurate perception of the visual cues of the landing environment.
This situation was illuminated in the NTSB report of a hard landing in Tampa on June 21, 2021, involving a Bell 407. Pilots were conducting night-vision-goggle (NVG) autorotations at the time and were well acquainted with the runway used for their practice. The accident pilot stated he had conducted “hundreds” of NVG autorotations to this runway in the past. However, prior to the night of the accident, the runway had recently been repaved and was much darker in color. As such, the visual cues the pilot was accustomed to had changed, and most likely affected his ability to judge the helicopter’s height and speed above the ground, which resulted in a higher-than-normal flair, low rotor rpm, and subsequent hard landing.
The flare will also differ greatly with changes in density altitude. This was especially apparent to the author during site visits to flight schools in Florida, Hawaii, Montana, and Utah to participate in recurrent training in autorotations. At higher density altitudes, with less dense air, there is less drag on the rotor blades. With the collective fully lowered, the rotor RPM will be faster at high density altitudes than at low density altitudes.
The rotor RPM with a fully lowered collective may be high enough to exceed the power-off limitations. A slight amount of collective pitch may be needed to maintain the rotor RPM within limits. According to helicopter flight test expert Shawn Coyle, this is especially true on rotor systems with more than two rotor blades. “It may not ever be possible to fully lower the collective,” he says.
Other effects on an autorotation performed at high density altitude include a higher rate of descent, reduced rotor RPM build in autorotation, low initial rotor RPM response, the requirement for a higher flare height and reduced engine performance for the go-around. The difference in the characteristics of the autorotation were significant compared to sea level. These last two points illustrate the importance of having an exposure to a wide variety of environmental conditions during autorotation proficiency training.
Full Touchdown Practice
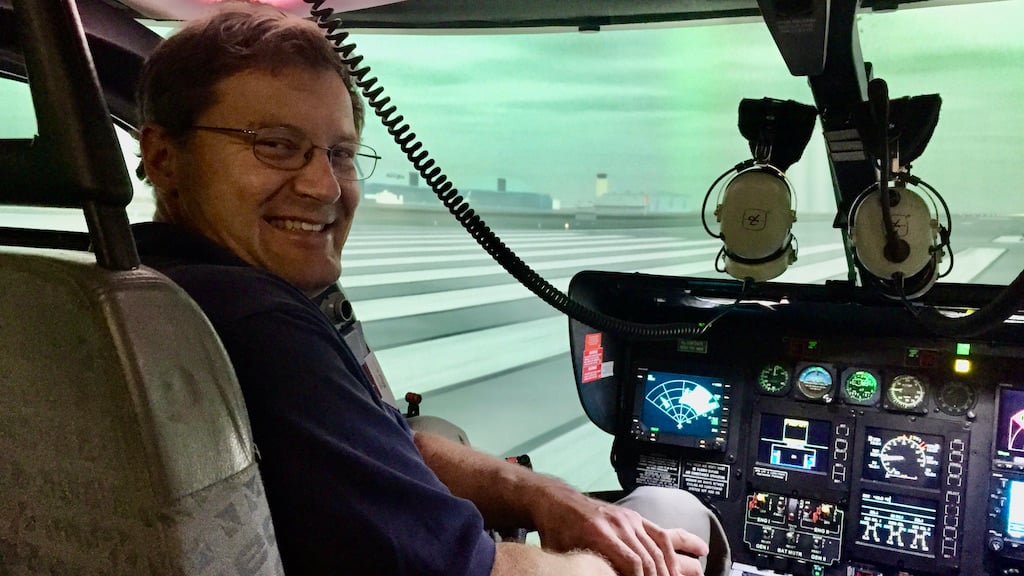
The majority of in-flight training autorotations are terminated with a power recovery to a hover. The FAA Airman Certification Standards (ACS) do not require applicants for the private, commercial or ATP certificates to demonstrate proficiency in full touchdown autorotations. Neither does 14 CFR 135 during initial and recurrent training. However, the Flight Instructor ACS do require a CFI applicant to demonstrate proficiency in full-touchdown autorotations.
The US Helicopter Safety Team’s (USHST) “Airmanship Bulletin: Full Touchdown Autorotation Training” highlights the pros and cons of full touchdown training. Advocates for full touchdown training believe the benefit the pilot receives in experiencing an autorotative landing and the opportunity to build proficiency in the technique greatly increases pilot confidence, thus reducing the change of a catastrophic outcome to a real engine failure. The team also believe that the power recovery aspect of the autorotation does not resemble the real situation and may even build a false sense of security on the part of the pilot.
In comparison, advocates for power-recovery claim that the increased risk of damaging the rotorcraft with the full touchdown maneuver is not worth the benefit gained over a power recovery to the hover. They also believe that with the increased reliability of today’s modern engines, the industry would damage more rotorcraft practicing for an event that rarely occurs. The USHST’s Airmanship Bulletin does not take either side in this debate.
With the lively debate on the pros and cons of full touchdown autorotation practice, it is worth asking about the prevalence of this maneuver within the data sample. It is perhaps surprising that there was only one accident. The NTSB attributed it to the pilot’s improper landing flare during a full-down autorotation. A lower-than-normal rotor rpm, faster-than-normal ground run speed, and the subsequent loss of directional control led to a runway excursion and roll-over.
Inadvertent Throttle Movements
On Feb. 18, 2020, near Tampa, the flight instructor in an Aerospatiale AS350 directed the student to conduct an autorotation with a 180° turn, followed by a power recovery. Abeam the departure end of runway, the instructor moved the throttle lever from the “fly” position to idle. While conducting the maneuver, the trainee overshot the runway and aligned the helicopter with the parallel taxiway.
Upon realizing that the helicopter was not in the correct position, the pilots chose to abort the maneuver and perform a go- around. While the pilot continued to fly the helicopter, the instructor inadvertently moved the throttle lever from idle aft toward the “off” position and then forward to the “fly” position. The engine experienced a total loss of power, and the instructor adjusted the throttle to no avail. The instructor took the controls, conducted a power-off autorotation, and landed the helicopter on the taxiway. The helicopter skidded about 180 ft., departed the taxiway onto adjacent grass, and came to rest in a drainage ditch.
The NTSB determined that the flight instructor’s inadvertent throttle reduction below idle resulted in a total loss of engine power and subsequent impact with terrain following a power-off autorotation.
The FAA has issued Special Airworthiness Bulletin SW-12-12, “Conducting Engine-Failure Simulation in Helicopters with Reciprocating Engines.” The bulletin cautions owners and operators of Schweizer 269C and 269C-1 helicopters to avoid throttle chops to full idle to minimize the possibility of engine stoppage.
Robinson Helicopter’s Safety Notice SN-38 (dated July 2003 and revised in October 2004), “Practice Autorotations Cause Many Training Accidents,” provides similar recommendations. It states: “do not roll throttle to full idle. Reduce throttle smoothly for a small visible needle split, then hold throttle firmly to override governor. Recover immediately if engine is rough or engine RPM continues to drop.”
Handling Oscillations
There are various flight conditions in which a helicopter will experience significant oscillations that can be difficult to control, and the recommended action is to deliberately place the helicopter into an autorotation. For example, the Robinson R22 flight manual instructs pilots to perform an autorotation in the event of unanticipated right yaw or to be prepared for an autorotation in the event of a malfunction of the clutch.
The point is that unlike autorotation practice in which the training maneuver begins from a stable condition (and no surprise), these events occur suddenly and create immense pitch, yaw and/or roll oscillations. Furthermore, these produce a cacophony of somatosensory illusions that can interfere with a pilot’s ability to focus vision and make accurate, fine-tuned muscle movements.
An example of the latter situation occurred to the pilot of a Eurocopter EC-130 while conducting a sightseeing flight near Kalapana, Hawaii, on March 5, 2020. The pilot detected the onset of a significant, high frequency vibration. As the helicopter slowed, the 7,878-hour pilot raised the collective and applied right tail rotor pedal, but the nose of the helicopter veered to the left. The pilot eventually applied full right tail rotor pedal, but the nose of the helicopter continued to the left.
About 200 ft. above ground level, with the right tail rotor pedal fully depressed, the helicopter began to spin to the left. The pilot attempted to stop the spin to no avail and eventually closed the throttle and performed a hovering autorotation. Two occupants received minor injuries and four others were uninjured. Post-accident investigation found a fracture in a tail rotor blade in the fenestron.
Clearly there is no safe method to replicate these abnormal motions in flight training. Unless a pilot has access to a flight training device or simulator that can accurately replicate these malfunctions and provide the sensory “wild ride,” it is entirely likely that a pilot will have no hands-on training in the proper response to these emergencies.
Fuel Mismanagement Accidents
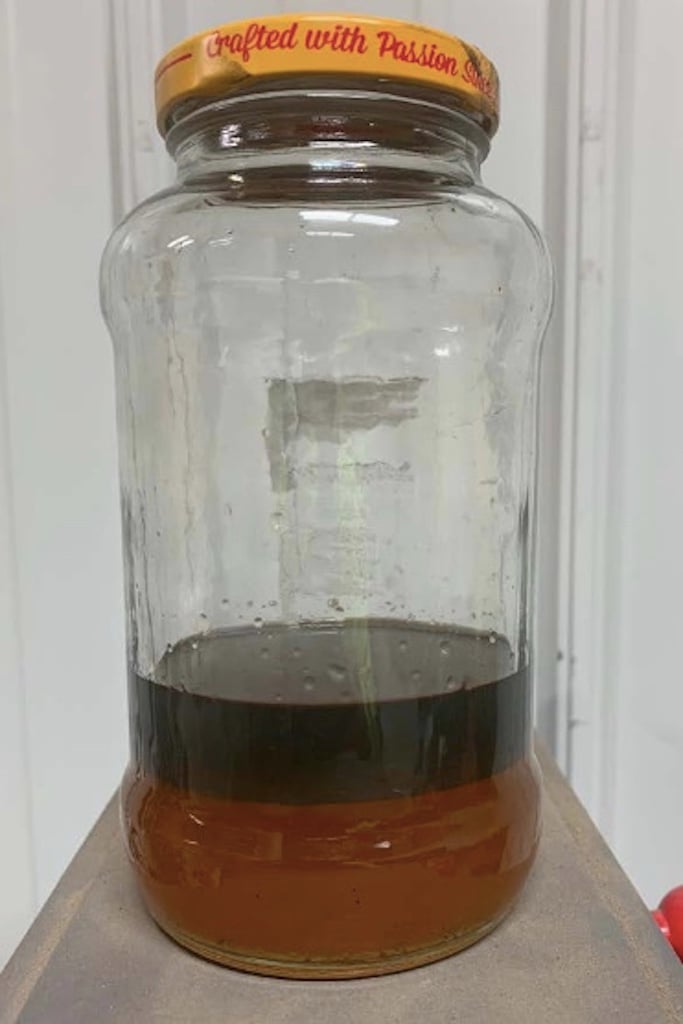
The pilot of a Robinson R44 was conducting agricultural application flight operations on July 27, 2020, near Hebron, Nebraska. During the initial climb-out, the pilot detected a power loss and initiated an autorotation into a cornfield. Substantial damage resulted to the aircraft’s fuselage, tail rotor and horizontal/vertical stabilizers.
Fuel drained by accident investigators from the gascolator, or fuel strainer, revealed a large quantity of water. On the evening prior to the accident, over 4 in. of rainfall had occurred at the airport. A fuel cap may have inadvertently been left off a fuel storage tank, allowing water to enter the tank. The operator admitted that rushing contributed to loading unfiltered fuel into the helicopter. The pilot did not sump the helicopter’s fuel tanks as required by the flight manual. The NTSB determined the loss of engine power was caused by water contamination in the fuel system.
These self-inflicted errors from fuel mismanagement, which included either fuel contamination or fuel exhaustion, occurred in seven accidents in the data sample. To put this into perspective, roughly 10% of the autorotation accidents in the four-year period could have been prevented through proper fuel management procedures.
Importance Of Realistic Training
The NTSB’s investigation of the accident emphasized the importance of realistic autorotation training in all environmental conditions. However, in-flight training of autorotations requires tightly controlling as much risk as possible. This includes no “surprise” simulated engine failures, and not practicing the maneuver in less than “good” conditions.
The problem is that in the real world, an autorotation can occur at any moment without warning and during environmental conditions that are adverse, such as experienced by aerial firefighting helicopters near a wildfire. In-helicopter autorotation training has the further disadvantages of being time-consuming and expensive.
The author’s hands-on experiences in the EC-135 Level D full-motion simulator at Metro Aviation’s Training Center in Shreveport, Louisiana, and the Loft Dynamics (formerly VRM Switzerland) H-125 flight training device in Zürich, Switzerland, explored the realism to practice abnormal flight conditions, including autorotations.
The advantages of a simulator are clearly evident during the practice of emergency procedures. Simulators allow a demonstration of an ideal maneuver as well as how not to do the maneuver, presenting common errors and ways to avoid them. Dozens of emergency maneuvers can be performed during a two-hour training session, many more than could be done in an actual rotorcraft in the same time frame. Risk-free practice of these maneuvers for variations in rotorcraft weight, density altitude, wind speed, and direction show how each factor will individually or in combination affect performance of an autorotation.
How do we provide sufficient training to pilots to make certain they are proficient in the complex emergency procedures in a rotorcraft under a variety of real-world conditions?
The NTSB’s “Safety Through Helicopter Simulators” safety alert provides a clear recommendation. “Consistent, standardized simulator training will help prepare pilots for the unexpected and will decrease the risk of an accident,” the safety board says. “Simulators can be a helpful tool for operators to provide pilot training on autorotations during any phase of flight, which reinforces the immediate responses required during actual emergencies.”
On a final note, Claude Vuichard demonstrated his modification to autorotation flares during our visit at Loft Dynamics. He demonstrated in the H-125 flight training device the advantages of his proposed modification while landing on a steep mountain slope. “Thought provoking” was my reaction.
Vuichard recently introduced the new technique to helicopter operators in the Alps. Could his proposed method help to reduce autorotation accidents? Given the credibility of his contributions with vortex ring state, his proposal for autorotation recovery is worth a full vetting from the noteworthy authorities in helicopter training, safety, human factors and aerodynamics.