Human Mars Lander Must Break New Ground
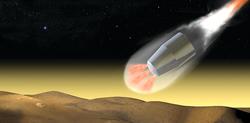
Credit: Karl Edquist/NASA Langley Research Center
For all the attention focused on how hard it will be to keep astronauts alive while they fly from Earth to Mars, the challenge of setting them safely down on the Martian surface will be just as difficult. Entry-descent-and-landing (EDL) experts who spoke at a Humans To Mars symposium here say the...
Subscription Required
This content requires a subscription to one of the Aviation Week Intelligence Network (AWIN) bundles.
Schedule a demo today to find out how you can access this content and similar content related to your area of the global aviation industry.
Already an AWIN subscriber? Login
Did you know? Aviation Week has won top honors multiple times in the Jesse H. Neal National Business Journalism Awards, the business-to-business media equivalent of the Pulitzer Prizes.