'Certifiable Trust' Required To Take Autonomous Systems Past 'Unmanned'
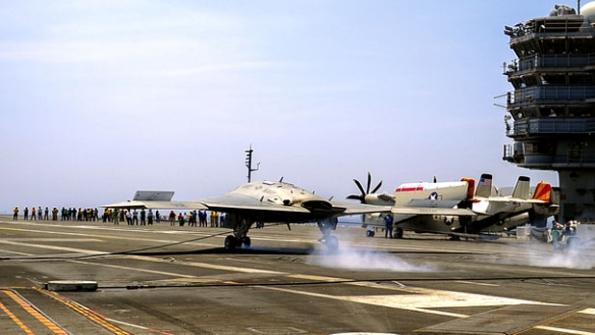
Credit: U.S. Navy
Aviation has been built around humans since before the origins of powered flight, but unmanned technology is opening new design spaces in unexpected ways. Now shaped by the strengths and weaknesses of pilots and controllers, how aircraft are flown and air traffic managed could change dramatically in...
Subscription Required
This content requires a subscription to one of the Aviation Week Intelligence Network (AWIN) bundles.
Schedule a demo today to find out how you can access this content and similar content related to your area of the global aviation industry.
Already an AWIN subscriber? Login
Did you know? Aviation Week has won top honors multiple times in the Jesse H. Neal National Business Journalism Awards, the business-to-business media equivalent of the Pulitzer Prizes.