Aviation Week Evaluates Boeing 787
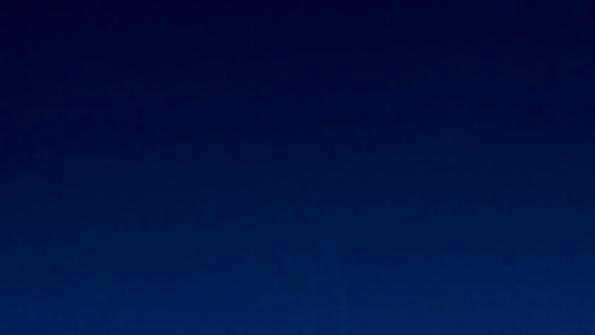
Credit: Boeing
Pilots who strap into Boeing's new 787 most likely will conclude that it is the easiest to fly and most intuitive jetliner ever built by the Seattle-based manufacturer, based upon our findings during a demo flight in mid-November. Five large-format LCD screens have 40% more display area than those...
Subscription Required
This content requires a subscription to one of the Aviation Week Intelligence Network (AWIN) bundles.
Schedule a demo today to find out how you can access this content and similar content related to your area of the global aviation industry.
Already an AWIN subscriber? Login
Did you know? Aviation Week has won top honors multiple times in the Jesse H. Neal National Business Journalism Awards, the business-to-business media equivalent of the Pulitzer Prizes.